Oluwole, O. R, O.;1 lalemi, A. O.;2 & Akinyele, B. J.3
1Department of General Studies, Federal College of Agriculture, Akure, P.M.B 724, Akure, Ondo State, Nigeria
2Department of Microbiology, Federal University of Technology, P.M.B 704, Akure, Ondo State, Nigeria
*Corresponding Author Email:omowunmi.oluwole@gmail.com
Abstract
The effect of Pleurotus ostreatus culture broth on the growth of toxigenic fungi, Aspergillus parasiticus and A. flavus, was studied. Its effect on the nutritional composition of compounded fish feed was also investigated. The mycelium of P. ostreatus was cultivated on potato dextrose agar for 17 days, and its antifungal activity against Aspergillus spp. was studied over 8 days. Bioactive compounds produced by P. ostreatus inhibited the growth of A. flavus and A. parasiticus. Three different fish feed formulations were prepared: the compounded fish feed only (C), the compounded fish feed plus P. ostreatus (CM), and a commercially obtained fish feed (COMM). The addition of P. ostreatus broth to the compounded fish feed for 4 days improved the protein and fibre contents of the compounded feed. The growth of catfish (Clarias gariepinus) fed the three feed formulations increased over the 31-day experimental period, with no significant difference at p > .05. However, the catfish fed with compounded feed plus P. ostreatus exhibited better growth performance (1.358 g mean weight gain) than the fish fed with the commercial feed (1.079 g mean weight gain). Overall, P. ostreatus demonstrates significant potential as a cost-effective antifungal agent because its cultivation requires minimal investment and risk, and it is a rich source of protein for application in the fish feed industry.
Keywords: Antifungal activity, aquaculture sustainability, Pleurotus ostreatus, Aspergillus spp., fish feed.
1. Introduction
Pleurotus ostreatus is a basidiomycete commonly found in temperate and tropical regions. It has been reported to be rich in protein and to contain bioactive compounds with antimicrobial properties (Lesa et al., 2022; Gafforov et al., 2023). This edible fungus, which belongs to the class Agaricomycetes (Krupodorova et al., 2024), is the second most cultivated fungus worldwide for commercial purposes, including food, medicine, and bioremediation (Correa et al., 2016; Shevchuk et al., 2023). Cultivating P. ostreatus is relatively easy because it grows more quickly than many other edible fungi and does not require complex growth conditions (Lesa et al., 2022). It adapts to a wide range of temperatures and humidity, requiring minimal investment, risk, and labour. Moreover, it can be grown on agricultural wastes such as corn husk, cereal straw, and wood sawdust, thereby contributing to environmental waste reduction (Sow & Ranjan, 2021). The use of P. ostreatus as an active ingredient in fish feed can boost the income of rural farmers who supply it for fish feed production, while also serving as a cheap source of protein (Gupta et al., 2018).
In addition, the mycelium of P. ostreatus has proven useful in the food, applied chemistry, bioscience, and medical industries. Products derived from its mycelium are ecofriendly and have positive effects on human health (Krupodorova et al., 2024). Several bioactive compounds obtained from P. ostreatus are useful as inhibitors of pathogenic organisms, in the prevention of diabetes, cancerous tumours, and as antioxidants (Krupodorova et al., 2024). According to Toros et al. (2023), the bioactive compounds from the mycelium of P. ostreatus possess antimicrobial and prebiotic properties (e.g., glucans, chitin, phenols, and terpenoids) that support the proliferation of beneficial gut microbiota while inhibiting the growth of harmful microorganisms, reducing infections and the frequent use of antibiotics. Application of P. ostreatus in feed production is a promising alternative to the use of antibiotics, reducing the risk of antibiotic resistance (Cruz-García et al., 2022; Toros et al., 2024).
In aquaculture, fish feed is formulated to ensure that the protein and energy contents support growth and yield more profit (Ashley-Dejo et al., 2022). According to Pascual et al. (2018), the mycelium of P. ostreatus improved the nutritional composition of fish feed ingredients, resulting in a significant increase in the growth of fish fed with the treated feed compared to controls. Similarly, Yerima et al. (2020) stated that the addition of the mycelium of the mushroom Ganoderma lucidium as a feed additive improved the protein quality of red hybrid tilapia. The inclusion of mushrooms, particularly P. ostreatus, proves useful in enhancing the nutritional component of fish feed and may serve as an alternative to antibiotics due to its antimicrobial effects and its role as a rich source of nutrients (Singhal et al., 2019). Aflatoxins, produced by toxigenic fungi such as Aspergillus spp. in nuts, cereals, and spices (in the field or in storage), have long been a major problem in the feed industry (WHO, 2018). These crops, which form a major ingredient of feed, become contaminated with toxins that reduce the growth performance of fish (Mwihia et al., 2018). The ability of P. ostreatus to produce bioactive compounds that antagonise toxigenic fungi may consequently prevent aflatoxin production (Marwa et al., 2019). In view of these benefits and the recent applications of P. ostreatus in various industries, this study aimed to assess (a) the antifungal potential of P. ostreatus mycelium against toxigenic fungi commonly contaminating cereals (Guan et al., 2021), (b) its effect on the nutritional composition of fish feed, and (c) the impact of the formulated fish feed on the growth of juvenile catfish (Clarias gariepinus).
2. Material and Methods
2.1 Collection of Samples
Fish feed ingredients (soya cake, maize, and groundnut cake) were obtained from four feed mills within the Akure metropolis, Ondo State, Nigeria. They were transported in clean polythene bags to the laboratory for microbiological analysis. Contamination was prevented by keeping the feeds in sealed polythene bags during transport.
The pure spawn of Pleurotus ostreatus was obtained from the Forestry Research Institute of Nigeria (FRIN) and transported in a sterile bottle to the laboratory for culturing.
2.2 Culturing and Identification of P. ostreatus
The pure spawn of P. ostreatus was cultured on potato dextrose agar (PDA) with chloramphenicol (250 mg/200 mL) incorporated to inhibit bacterial growth, for 4 days at ambient temperature. Morphological identification was conducted according to Khan et al. (2011) by observing the colour and growth pattern of the mycelium.
2.3 Isolation and Identification of Toxigenic Fungi in Fish Feed Ingredients
The toxigenic fungi, A. flavus and A. parasiticus, were isolated from the feed ingredients by the pour plate method on PDA. Plates were incubated for 3 days at ambient temperature after plating 1 mL of the first and second dilutions. Pure isolates were obtained by streaking three times on fresh PDA. Identification was performed morphologically according to Pitt and Hocking (1997) by observing the colour of the spores on PDA and the structures of the conidia and conidiophores under a light microscope.
2.4 Detection of Aflatoxins
Detection of aflatoxins G1, G2, B1, and B2 in A. flavus and A. parasiticus was performed using a Shimadzu Prominence system equipped with an ultraviolet–visible spectrophotometer (UV-VIS) detector (SPD-20AV) at a wavelength of 365 nm. Calibration was done using mixed standards of aflatoxins at known concentrations. Aflatoxins in the Aspergillus spp. biomass were separated on an HPLC column using a mobile phase of water, methanol, and acetonitrile (50:40:10, v/v) at a flow rate of 0.8 mL/min at 34°C. Aflatoxins were identified by matching sample peak retention times with those of standards (Schuster et al., 1996).
2.5 Antagonistic Activity of P. ostreatus against A. flavus and A. parasiticus
The antifungal activity of P. ostreatus was assessed by inoculating 0.5 mL of 2-day-old spore suspensions of A. parasiticus and A. flavus (10^8 sfu/mL) and 20 µL of a 17-day-old cell suspension of P. ostreatus (0.38 mycelial turbidity at 640 nm) onto the centre of each plate (using a 6-mm-diameter well). The inoculated plates were incubated for 8 days, and the zone of inhibition was recorded using the agar well diffusion method (Marwa et al., 2019).
2.6 Detection of Metabolites of P. ostreatus Using GC–MS
Metabolites of P. ostreatus were detected using a GC–MS-QP2010 PLUS Shimadzu system with a capillary column (30 m × 0.25 mm, film thickness 0.25 µm) coated with 95% dimethyl polysiloxane. Helium was used as the carrier gas at a flow rate of 0.5 mL/min, with a 1 µL injection volume. The inlet temperature was set at 250°C, and the oven was initially held at 80°C for 4 min, then increased to 200°C. The total run time was 25 min. The mass spectrometry transfer line was maintained at 180°C. GC–MS analysis was conducted using electron impact ionisation at 70 eV, and data were evaluated using total ion count (TIC) for compound identification and quantification. The spectra of components were compared with those in the NIST (2014) GC–MS library (Karimi & Jaafar, 2011).
2.7 Preparation of Fish Feed
Fish feed was prepared using the Pearson square method to obtain 40% crude protein, using soya cake (19.5%), maize (30%), groundnut cake (16.5%), fish meal (20%), limestone (1%), salt (0.5%), bonemeal (1%), binder (2%), vegetable oil (2.5%), vitamin premix (1%), mineral premix (1%), and wheat offal (5%) (Arsenos et al., 2023). The compounded fish feed was sterilised in an oven at 110°C for 2 hours.
For the second formulation, 3.5 mL of a 17-day-old cell suspension of P. ostreatus (0.38 mycelial turbidity at 640 nm) was added to 500 g of the sterilised compounded fish feed for 4 days. One hundred and fifty millilitres of sterile distilled water was added to ensure proper mycelial ramification (Ibitoye et al., 2020). The compounded fish feed only (C; control) and the compounded fish feed with P. ostreatus (CM) were pelletised into 2-mm-diameter pellets using a spaghetti pelletising machine at the fish farm of the Federal University of Technology, Akure, Nigeria, and then sun-dried.
The third formulation consisted of 500 g of commercially sold 2-mm fish feed (COMM; control).
2.8 Feeding Clarias gariepinus with Formulated Diets
Juvenile catfish (Clarias gariepinus, n = 30; approximately 8 weeks old) were obtained from the fish farm at the Federal University of Technology, Akure, Ondo State, Nigeria. The fish were acclimatised for 3 days in groups of 10 per tank (dimensions: 41 × 29 × 27 cm; 40-L capacity, filled with 30 L of clean water) at ambient temperature. During acclimation, fish were fed twice daily at 09:00 h and 16:00 h with commercial feed, as per the farm’s routine. The use of the fish was in accordance with international standards of animal welfare. During the experiment and acclimation, water was changed every 3 days. After water changes, the fish were monitored for 18 hours and showed no signs of ill health. Each group was then fed approximately 2 g of one of the diet treatments (COMM, C, or CM) daily by feeding twice daily (09:00 h and 16:00 h) for the next 31 days. The average weight of each group was monitored weekly (Montes de Oca et al., 2024).
2.9 Measurement of Protein, Carbohydrate, And Fibre Content of the Fish Feed Formulations
The proximate analysis of the fish feed formulations was carried out in triplicate to determine the protein, fibre, and carbohydrate contents. Crude protein was estimated using the Kjeldahl method; total nitrogen was multiplied by a protein factor of 6.25. Crude fibre was determined by washing samples in 1.25% acid and base for 30 minutes each at 100°C and then oven-drying at 105°C until a constant weight was achieved. Total carbohydrate was calculated by subtracting the sum of the percentages of moisture, crude protein, lipid, ash, and crude fibre from 100.
CHO (%) = {100 – (moisture + crude protein + crude lipid + ash + crude fibre)} (AOAC, 2000).
2.10 Statistical Analysis
Analysis of variance (ANOVA) was used to analyse the data at p < .05 using IBM SPSS version 23 to compare significant differences in the protein, fibre, and carbohydrate contents of the formulated fish feeds. A post hoc test (Duncan test) was used to separate the means.
3. Results
3.1 Total Fungal Count in Fish Feed Ingredients
The total fungal counts in the fish feed ingredients varied among feed mills. Feed mill A recorded the highest fungal count for groundnut cake (9.1 × 10³ sfu/mL), whereas feed mill B had the lowest fungal count for groundnut cake (0.55 × 10² sfu/mL) (see Table 1).
Table 1: Total Fungal Count (sfu/mL) in Fish Feed Ingredients
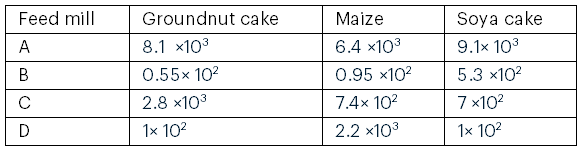
3.2 Morphological Characteristics of the Toxigenic Fungi in Fish Feed Ingredients
Table 2 shows the morphological characteristics of the isolated Aspergillus spp. The isolates were identified as Aspergillus flavus and Aspergillus parasiticus based on the colour of the spores on PDA and the structures of the conidia and conidiophores observed under a light microscope.
Table 2: Morphological and Microscopic Characteristics of Toxigenic Fungi in Fish Feed Ingredients
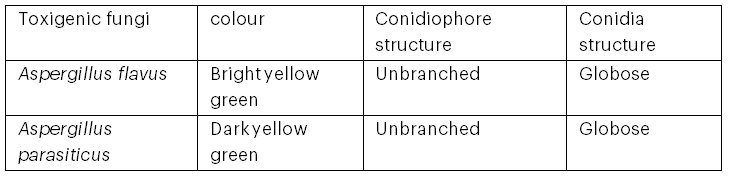
3.3 Morphological Characteristics of Cultured P. Ostreatus
The mycelium of P. ostreatus was white, cotton-like, and exhibited a tufted, fluffy appearance (see Plate 1).
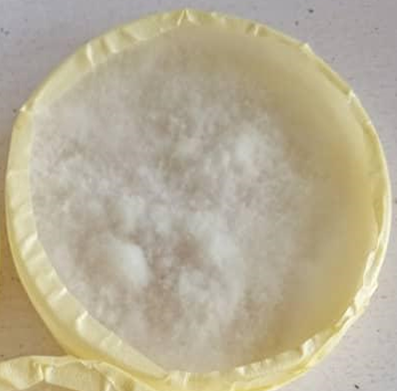
Plate 1: Mycelium of P. ostreatus on Potato Dextrose Agar
3.4 Detection of Aflatoxins
A. flavus produced aflatoxin B₁, which was quantified at 5.66 ppb, and A. parasiticus produced aflatoxins G₁ and G₂, quantified at 19.74 ppb and 2.96 ppb, respectively (see Figures 1 and 2).
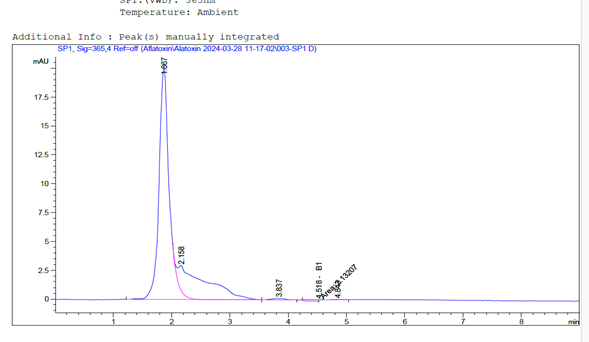
Figure 1: Chromatogram of Aflatoxin B₁ Produced by A. flavus
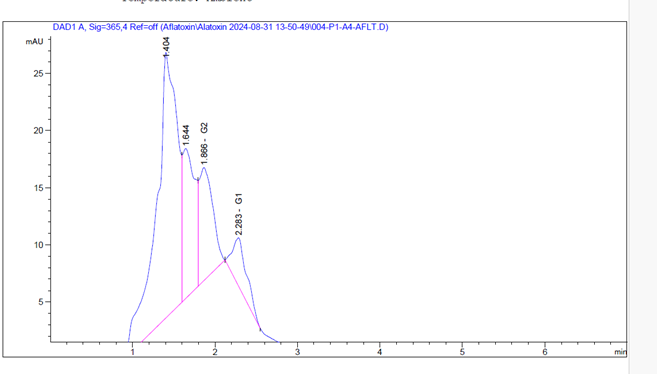
Figure 2: Chromatogram of Aflatoxins G₁ and G₂ Produced by A. parasiticus
3.5 Antifungal Potential of P. Ostreatus on A. Flavus and A. Parasiticus
Using the agar well diffusion method, the zone of inhibition was recorded. On the second day, the zone of inhibition for A. flavus was 24 mm and for A. parasiticus was 20 mm. On the third day, the zone of inhibition for A. flavus was 16 mm and for A. parasiticus was 17 mm. The inhibition zone was maintained until day 8 (see Plates 2 and 3).
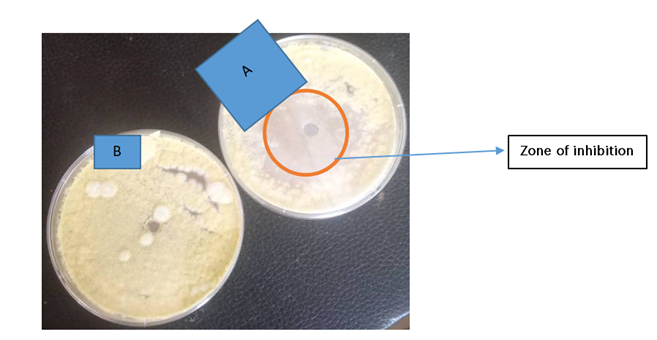
Plate 2: Antifungal Activity of P. ostreatus against A. flavus
- Experiment: P. ostreatus against A. flavus
- Control: A. flavus only
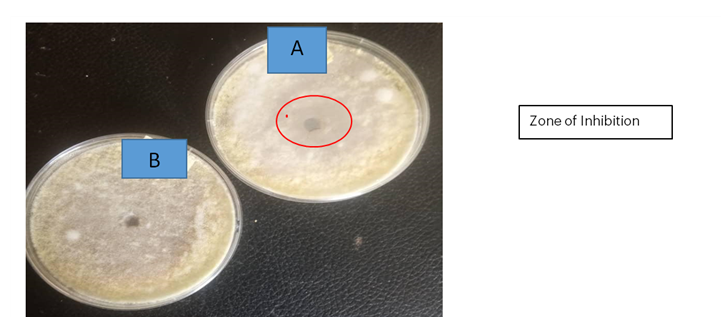
Plate 3: Antifungal Activity of P. ostreatus against A. parasiticus
- Experiment: P. ostreatus against A. parasiticus
- Control: A. parasiticus only
3.6 Metabolites of P. ostreatus Using GC–MS
Metabolites such as methyl esters, 11-tridecen-1-ol, hexadecanoic acid, squalene, and palmitic acid were detected in the P. ostreatus broth. Palmitic acid, methyl ester, was present at 11.92% of the peak area. These metabolites were identified as the antagonistic compounds against the moulds (see Figure 3).
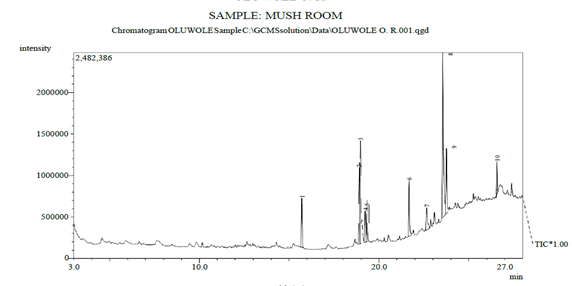
Figure 3: Spectrum of Metabolites Identified in P. ostreatus Broth
3.7 Proximate Composition of the Different Fish Feed Formulations
The protein content of the compounded fish feed with P. ostreatus was significantly higher than that of the two other fish feed formulations, and the fibre content of the fish feed with P. ostreatus was significantly higher than that of the commercial feed (see Table 3). The higher protein content in the compounded feed with P. ostreatus may be associated with the high protein content present in mushrooms (Pascual et al., 2018).
Table 3: Composition of Carbohydrate, Fibre, and Protein in Fish Feed Formulations
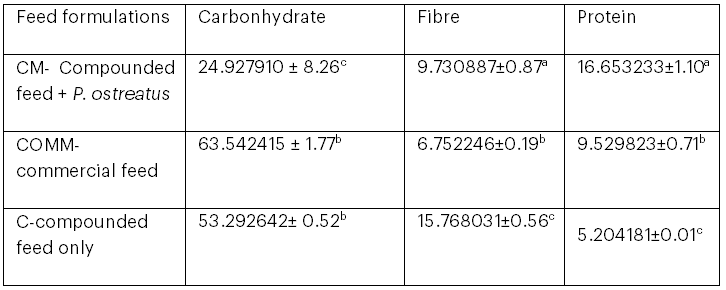
Statistical significance was set at p < .05. Means sharing the same superscript within each column are not significantly different.
3.8 Feeding Clarias Gariepinus with Formulated Diets
Figure 4 illustrates the weekly weight gain of catfish fed with commercial feed, compounded feed plus P. ostreatus, and compounded feed only. Fish fed with the compounded feed plus P. ostreatus gained more weight compared to those fed with the commercial feed.
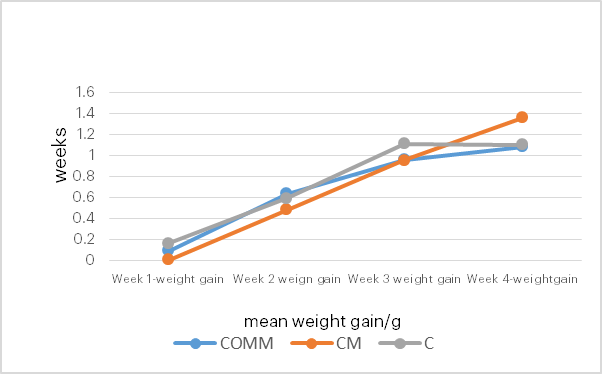
Figure 4: Weekly Mean Weight Gain of Fish Fed with Different Diets
C: Compounded feed only
COMM: Commercial feed
CM: Compounded feed plus P. ostreatus
4. Discussion
In this study, the P. ostreatus cell suspension inhibited the growth of toxigenic A. flavus and A. parasiticus in vitro. This finding is in agreement with Golak-Siwulska et al. (2018), who reported that the mycelium of P. ostreatus is a source of bioactive compounds such as terpenoids, fatty acid esters, and polyphenols. These bioactive compounds might be responsible for the antifungal activity of P. ostreatus. According to Ren et al. (2020), certain bioactive compounds produced by microorganisms are antagonistic to the growth of moulds. GC–MS data show that P. ostreatus mycelium produced methyl esters and acids, and palmitic acid, methyl ester (11.92%), exhibited antagonistic properties against moulds. The mycelial extracts of P. ostreatus have been found to produce antimicrobial compounds such as polysaccharides, terpenoids, steroidal glycosides, lipids, and their derivatives (Iwalokun et al., 2007; Patel et al., 2012; Lesa et al., 2022). These compounds inhibit the growth of pathogenic strains (e.g., Bacillus subtilis and Escherichia coli), with the antimicrobial activity depending on the extraction solvent used (Lesa et al., 2022). In this study, many of the compounds detected by GC–MS in the P. ostreatus broth belonged to terpenoids (e.g., squalene) and lipid derivatives (e.g., hexadecanoic acid and methyl esters). The ability of P. ostreatus bioactive compounds to inhibit the growth of A. flavus and A. parasiticus is particularly beneficial, as these Aspergillus spp. produce aflatoxins B₁, B₂, G₁, and G₂ in food and feed, either in storage or in the field (Pickova et al., 2021). Aflatoxins have been a significant concern in surveys covering 100 countries from 2008 to 2017 in rice and feed ingredients (Riahi et al., 2021), and contaminated commodities have a substantial economic impact (Shabeer et al., 2022). Consumption of these toxins causes liver damage, immunosuppression, and stunted growth in animals. The most effective measure to prevent aflatoxin production is to inhibit the growth of the producing organisms (Marwa et al., 2019). Although chemicals such as sodium bisulphite and calcium hydroxide have been used to inhibit Aspergillus spp. in foods, these methods are associated with nutrient degradation, high cost, and chemical residues (Pickova et al., 2021). An alternative method is the use of microorganisms such as lactic acid bacteria, yeast, and mushrooms, which avoids these problems. The use of P. ostreatus mycelium as a source of bioactive compounds to inhibit Aspergillus spp. represents a biological method to prevent aflatoxin production in food and feed; the use of probiotics or edible microorganisms such as P. ostreatus is increasingly sought after (Guan et al., 2021).
The data in this study show that juvenile catfish fed with compounded feed containing P. ostreatus exhibited a better increase in mean weight (from 0.004 g at week 1 to 1.36 g at week 4) compared with those fed with commercial feed (from 0.09 g at week 1 to 1.08 g at week 4). This finding is consistent with Salama et al. (2020), who reported that the use of P. ostreatus as a dietary additive improved growth in Nile tilapia, and with Singhal et al. (2019), who noted that P. ostreatus is nutritious and promotes fish growth. The proximate analysis in this study showed that the compounded feed with P. ostreatus had the highest protein content (16.65%) compared to the commercial feed (9.53%) and compounded feed only (5.20%), and its fibre content (9.73%) was higher than that of the commercial feed (6.75%). This is in agreement with Lesa et al. (2022), who reported that P. ostreatus is highly nutritious, containing high levels of protein, fibre, minerals, and vitamins. The high protein content required for optimal growth of juvenile catfish is approximately 40–50% (Langi et al., 2024). The potential of P. ostreatus to increase the protein content of fish feed may be due to its high crude protein level (approximately 72%–83%; Gupta et al., 2018; Lesa et al., 2022). Protein from edible mushrooms, including P. ostreatus, is easily digestible and contains all the essential amino acids, in contrast to proteins from animal or plant sources (Ayimbila & Keawsompong, 2023). Moreover, mushroom-derived proteins possess antimicrobial properties and can be used to fortify food and feed (Ayimbila & Keawsompong, 2023). According to Aderolu et al. (2018), the ratio of protein and energy sources such as carbohydrates and lipids must be in the correct proportion to support fish growth. The improved growth of catfish fed with compounded feed with P. ostreatus (1.36 g weight gain) compared with commercial feed (1.08 g weight gain) in this study suggests that the nutrient ratios in the compounded feeds are optimal for fish growth, and that P. ostreatus is an effective source of protein, fibre, carbohydrates, and vitamins.
5. Conclusion
The findings of this study establish that P. ostreatus can inhibit the growth of A. flavus and A. parasiticus and enrich compounded fish feed with a higher protein content. Therefore, P. ostreatus can be applied in the fish feed industry both as a cost-effective protein source and as an inhibitor of aflatoxin-producing fungi. P. ostreatus-fortified food and feed have the potential to improve human and animal health by enhancing fish growth and increasing the income of fish farmers. However, large-scale application of P. ostreatus in feed production may be limited by the availability of readily obtainable substrates for mushroom cultivation.
Acknowledgement
The authors are grateful to the Department of Microbiology, School of Life Sciences, The Federal University of Technology, Akure, Ondo State, Nigeria, for providing appropriate support in terms of equipment and laboratory facilities. The available laboratory equipment included an oven, microscope, and autoclave.
Funding
The authors declare that no funds, grants or other support were received during the preparation of this manuscript.
Authors’ Contributions
Material preparation and data collection were carried out by Oluwole O. R., and analysis was performed by Oluwole O. R. The first draft of the manuscript was written by Oluwole O. R., Olalemi A. O., and Akinyele B. J.
Conflict of interest
The authors have no relevant financial or non-financial interests to disclose
References
Aderolu, A. Z., Lawal, M. O., Awobajo, F. O., Olaniyan, S., & Bello, Y. (2018). Dietary energy requirement of Clarias gariepinus juveniles at fixed crude protein and its effects on growth, nutrient performance, haematology and biochemical indices. Animal Research International, 15(3), 3090–3100.
AOAC. (2000). Official methods of analysis (17th ed.). Association of Official Analytical Chemists, Washington, DC, 2200, pp. 324–329.
Ashley-Dejo, S. S., Musa, M. A., Ogah, I. S., Sabo, M., & Ogo, M. P. (2022). Oyster mushroom meal as partial replacement of maize meal in diets of Nile tilapia (Oreochromis niloticus). Livestock Research for Rural Development, 34, Article #80. Retrieved November 26, 2024, from http://www.lrrd.org/lrrd34/9/3 480ashl.html
Ayimbila, F., & Keawsompong, S. (2023). Nutritional quality and biological application of mushroom protein as a novel protein alternative. Current Nutrition Reports, 12(2), 290–307. https://doi.org/10.1007/s13668-023-00468-x
Corrêa, R. C. G., Brugnari, T., Bracht, A., Peralta, R. M., & Ferreira, I. C. (2016). Biotechnological, nutritional and therapeutic uses of Pleurotus spp. (oyster mushroom) related with its chemical composition: A review on the past decade findings. Trends in Food Science & Technology, 50, 103–117.
Cruz-García, L. F., Ponce-Palafox, J. T., Hernández-Hernández, L. H., Tello-Salgado, I., Hernández-Ocampo, D., & Benítez-Mandujano, M. A. (2022). Effect of mushroom (Pleurotus djamor var. roseus) meal as feed supplement on the haematological responses and growth performance of Nile tilapia (Oreochromis niloticus) fingerlings. Latin American Journal of Aquatic Research, 50(1), 13–21.
Golak-Siwulska, I., Kałużewicz, A., Spiżewski, T., Siwulski, M., & Sobieralski, K. (2018). Bioactive compounds and medicinal properties of oyster mushrooms. Folia Horticulturae, 30(2), 191–201.
Gupta, S., Summuna, B., Gupta, M., & Annepu, S. K. (2018). Edible mushrooms: Cultivation, bioactive molecules, and health benefits. In Bioactive molecules in food (Vol. 1, pp. 1–33).
Ibitoye, O. A., Olaniyi, O. O., Ogidi, C. O., & Akinyele, B. J. (2020). Lactic acid bacteria bio-detoxified aflatoxins contaminated cereals, ameliorate toxicological effects and improve haemato-histological parameters in albino rats. Toxin Reviews. https://doi.org/10.1080/15569543.2020.1817088.
Iwalokun, B. A., Usen, U. A., Otunba, A. A., & Olukoya, D. K. (2007). Comparative phytochemical evaluation, antimicrobial and antioxidant properties of Pleurotus ostreatus. African Journal of Biotechnology, 6(15).
Karimi, E., & Jaafar, H. Z. (2011). HPLC and GC–MS determination of bioactive compounds in microwave-obtained extracts of three varieties of Labisia pumila Benth. Molecules, 16(8), 6791–6805.
Khan, S. M., Nawaz, A., Malik, W., Javed, N., Yasmin, T., ur Rehman, M., … & Khan, A. A. (2011). Morphological and molecular characterization of oyster mushroom (Pleurotus spp.). African Journal of Biotechnology, 10(14), 2638–2643.
Krupodorova, T., Barshteyn, V., Tsygankova, V., Sevindik, M., & Blume, Y. (2024). Strain-specific features of Pleurotus ostreatus growth in vitro and some of its biological activities. BMC Biotechnology, 24(1), 9.
Langi, S., Maulu, S., Hasimuna, O. J., Kaleinasho Kapula, V., & Tjipute, M. (2024). Nutritional requirements and effect of culture conditions on the performance of the African catfish (Clarias gariepinus): A review. Cogent Food & Agriculture, 10(1), 2302642.
Lesa, K. N., Khandaker, M. U., Mohammad Rashed Iqbal, F., Sharma, R., Islam, F., Mitra, S., & Emran, T. B. (2022). Nutritional value, medicinal importance, and health‐promoting effects of dietary mushroom (Pleurotus ostreatus). Journal of Food Quality, 2022(1), 2454180.
Marwa, A. M., Ramsey, A. M., & Zohri, A. A. (2019). The action mechanism and biocontrol potentiality of novel isolates of Saccharomyces cerevisiae against the aflatoxigenic Aspergillus flavus. Letters in Applied Microbiology, 68(2), 104–111.
Mwihia, E. W., Mbuthia, P. G., Eriksen, G. S., Gathumbi, J. K., Maina, J. G., Mutoloki, S., Waruiru, R. M., Mulei, I. R., & Lyche, J. L. (2018). Occurrence and levels of aflatoxins in fish feeds and their potential effects on fish in Nigeria, Kenya. Toxins, 10(5), 543. https://doi.org/10.3390/toxins10120543
Montes de Oca, S., Téllez-Téllez, M., Arce, E., García-Rodríguez, J., Burciaga, L. M., & de Lourdes Acosta-Urdapilleta, M. (2024). Effect of mushroom powder (Pleurotus ostreatus and Pleurotus djamor) on skin coloration and inflammation in Siamese fighting fish (Betta splendens). Journal of Applied Aquaculture, 1–16.
Pascual, M. M., Hualde, J. P., Bianchi, V. A., Moreno, P., Castro, J. M., & Luquet, C. M. (2018). Wheat grains fermented by fungal mycelia (Pleurotus ostreatus or Lentinus edodes) as alternative feed ingredients for juvenile rainbow trout (Oncorhynchus mykiss). Aquaculture International, 26(6), 1339–1352.
Patel, Y., Naraian, R., & Singh, V. K. (2012). Medicinal properties of Pleurotus species (oyster mushroom): A review. World Journal of Fungal and Plant Biology, 3(1), 1–12.
Pickova, D., Ostry, V., Toman, J., & Malir, F. (2021). Aflatoxins: History, significant milestones, recent data on their toxicity and ways to mitigation. Toxins, 13(6), 399.
Pitt, J. I., & Hocking, A. D. (1997). Methods for isolation, enumeration and identification. In Fungi and food spoilage (pp. 21–57).
Ren, X., Zhang, Q., Zhang, W., Mao, J., & Li, P. (2020). Control of aflatoxigenic molds by antagonistic microorganisms: Inhibitory behaviours, bioactive compounds, related mechanisms, and influencing factors. Toxins, 12(1), 24.
Riahi, I., Pérez-Vendrell, A. M., Ramos, A. J., Brufau, J., Esteve-García, E., Schulthess, J., & Marquis, V. (2021). Biomarkers of deoxynivalenol toxicity in chickens with special emphasis on metabolic and welfare parameters. Toxins, 13, 217. https://doi.org/10.3390/toxins13030217
Salama, F. A., Farag, F. T., Tonsy, H. D., Abu-Zeid, M. Y., & Ibrahim, N. A. (2020). Use of by-products of mushroom (Pleurotus ostreatus) grown on rice straw in Nile tilapia (Oreochromis niloticus) fingerling diets. Egyptian Journal for Aquaculture, 10(3), 21–42.
Schuster, R., Marx, G., & Rothaupt, M. (1996). Analysis of mycotoxins by HPLC with automated spectroscopic confirmation by spectral library. Agilent Techno-logies.
Shabeer, S., Asad, S., Jamal, A., & Ali, A. (2022). Aflatoxin contamination, its impact and management strategies: An updated review. Toxins, 14, 307. https://doi. org/10.3390/toxins14050307
Shevchuk, Y., Kuypers, K., & Janssens, G. E. (2023). Fungi as a source of bioactive molecules for the development of longevity medicines. Ageing Research Reviews, 87, 101929.
Singhal, S., Rasane, P., Kaur, S., Garba, U., Singh, J., Raj, N., & Gupta, N. (2019). Mushroom cultivation, processing and value-added products: A patent-based review. Recent Patents on Food, Nutrition & Agriculture, 10(1), 3–19.
Törős, G., El-Ramady, H., Prokisch, J., Velasco, F., Llanaj, X., Nguyen, D. H., & Peles, F. (2023). Modulation of the gut microbiota with prebiotics and antimicrobial agents from Pleurotus ostreatus mushroom. Foods, 12(10), 2010.
Törős, G., El-Ramady, H., Béni, Á., Peles, F., Gulyás, G., Czeglédi, L., … & Prokisch, J. (2024). Pleurotus ostreatus mushroom: A promising feed supplement in poultry farming. Agriculture, 14(5), 663.
World Health Organization. (2018). Food safety digest: Aflatoxins (REF No: WHO/NMH/FOS/RAM/18.1).
Yerima, G. (2020). Performance of mycelial biomass from the mushroom Ganoderma lucidum as a feed additive on growth and quality of red hybrid tilapia (Oreochromis spp.) (Master’s thesis, University of Malaya, Malaysia).
About this Article
Cite this Article
APA
Oluwole, O. R, Olalemi, A. O. & Akinyele, B. J.(2025). Antifungal Potential of Pleurotus ostreatus and Its Application in Fish Feed for Clarias gariepinus. In Akinyele B.J., Kayode R. & Akinsemolu A.A. (Eds.), Microbes, Mentorship, and Beyond: A Festschrift in Honour of Professor F.A. Akinyosoye. SustainE
Chicago
Oluwole, O. R, Olalemi, A. O. and Akinyele, B. J. 2025. “Antifungal Potential of Pleurotus ostreatus and Its Application in Fish Feed for Clarias gariepinus.” In Microbes, Mentorship, and Beyond: A Festschrift in Honour of Professor F.A. Akinyosoye, edited by Akinyele B.J., Kayode R. and Akinsemolu A.A., SustainE.
Received
15 December 2024
Accepted
10 January 2025
Published
4 February 2025
Corresponding Author Email: omowunmi.oluwole@gmail.com
Disclaimer: The opinions and statements expressed in this article are the authors’ sole responsibility and do not necessarily reflect the viewpoints of their affiliated organizations, the publisher, the hosted journal, the editors, or the reviewers. Furthermore, any product evaluated in this article or claims made by its manufacturer are not guaranteed or endorsed by the publisher.
Distributed under Creative Commons CC-BY 4.0
Share this article
Use the buttons below to share the article on desired platforms.