Margaret Ekua Amosah 1,2 Deborah Asarebea Asare 1 Eugenia Anderson 1,3 Grace Ofori-Sarpong 1
1University of Mines and Technology, Tarkwa, Ghana, 03123
2Goldenstar (Wassa) Limited, Accra, Ghana
3Newmont Ghana Gold Limited P.M.B Airport Post Office, Accra, Ghana
*Corresponding Author Email: margaretekuaamosah@gmail.com …
Highlights
- Heavy metals have the potential to bioaccumulate in the environment since they are non-biodegradable
- Arsenic seeps into the environment through natural and manmade activities
- Contaminated soil has the potential of transporting toxic materials into the plants in its surroundings through xylems in plants’ roots
- Colocasia esculenta can remove arsenic, about 68 mg As/m2/day, from wetland environments.
- Care should be taken as to where Colocasia esculenta plants are planted, their source of irrigation, and the nature of the environment when grown as a source of food.
Graphical Abstract
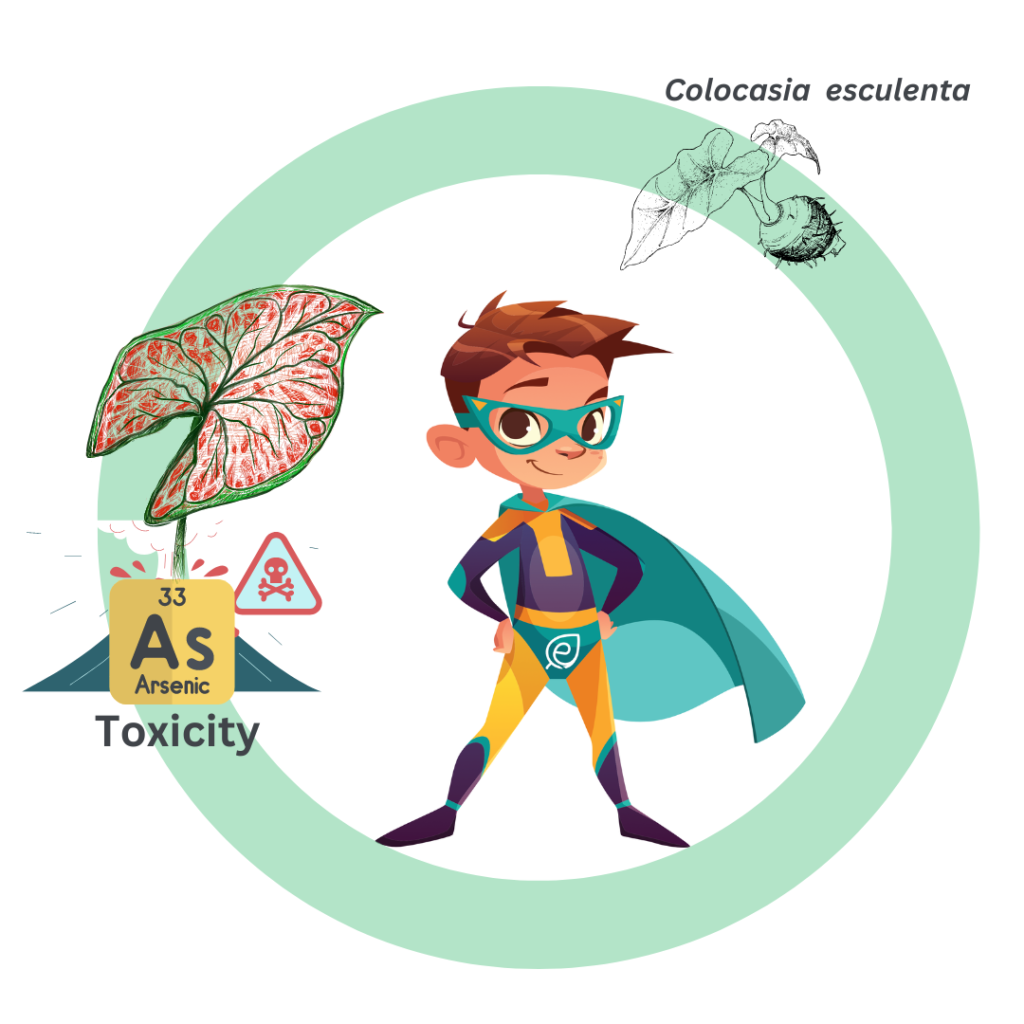
Abstract
The exposure of heavy metals such as mercury, cadmium, arsenic, chromium, thallium, and lead, and their interactions with soil and plants are of much concern due to their potential health effect. Phytoremdiation is one method used by plants to clean up soils contaminated with these heavy metals. Arsenic is a carcinogenic substance that thwarts plant growth and causes skin cancer in humans, among other health effects. Although arsenic decontamination by phytoremediation has been reported, the literature is silent about the toxicity and edibility of the plant after arsenic uptake. This study, therefore, assessed the toxicity of the Colocasia esculenta plant after using it as a phyto-sorbent for four weeks in a simulated arsenic-contaminated soil. The Bioaccumulation Factor (BAF) and the Translocation Factor (TF), calculated after the experiment, were 1.43 and 1.60 respectively indicating its hyper-accumulative property and high translocation of arsenic through the various parts. There was a decreasing trend of arsenic concentration in the solution samples across the weeks, and an accumulation of 58%, 28%, and 14% of arsenic in the stem, roots, and leaves of the plant respectively. The results showed that Colocasia esculenta is a good accumulator of arsenic from the soil. A comparison of the different arsenic levels recorded in the plant parts with standards, presents a great concern in consuming this plant exposed to an arsenic-contaminated environment. However, further studies should be conducted to ascertain the bioavailable state of arsenic in the plant parts after its uptake.
Keywords: Arsenic, Toxicity, Phytoremediation, Colocasia esculenta, Edibility.
1. Introduction
1.1 Arsenic Poisoning and the Environment
The utilization of heavy metals from the earth’s crust through human activities and its processing has resulted in the depositionof these metals on the surface of the earth. Heavy metals have the potential to bioaccumulate in the environment since they are non-biodegrade, hence increasing the chances of polluting food (Ali et al., 2019).
. Since heavy metals are dangerous, there is the need to prevent the risk and harm they bring, especially to living things found in the environment. Heavy metals are either carcinogenic, mutagenic, teratogenic, or endocrine disruptors causing disorder in neurons and changes in attitude especially in children (Mojiri et al., 2013; Ali et al., 2019). As explained by Arif et al. (2016) certain metal elements such as copper, iron, manganese, molybdenum, nickel, and zinc in the soil are necessary for the normal growth and metabolism of plants while other heavy metals like arsenic, cadmium, mercury, lead can easily prevent the healthy growth of plants since they are not responsible for any known physiological function in plants. The poisoning of food is mostly from the contaminated plant parts which are transferred to animals and humans through the food chain at higher concentrations greater than optimal levels required in the soil (Singh et al., 2011). Most plants take up heavy metals including arsenic, using their roots and are transferred into their stems and leaves where some are either lost, are broken down to less harmful states, or are permanently stored (Chibuike & Obiora, 2014).
Arsenic is a naturally occurring heavy metal present in the air, soil, and water used as an industrial alloying agent, as well as in the production of glass, pigments, textiles, paper, metal adhesives, wood preservatives, pesticides, and feed additives. It seeps into the environment through both natural and manmade activities. Jang et al. (2016) explain that sources of natural exposure include weathering of rocks, volcanic activity, and windblown dust while anthropogenic activities of man include electroplating, gas exhaust, smelting of metals, combustion of fuels, pesticides usage, wood preservative, and acid mine drainage (AMD). Arsenic can be grouped into organic arsenic compounds (such as those found in seafood) which are relatively safe and inorganic arsenic compounds (such as those found in water) which is a known environmental toxin and may exist as arsenite and arsenate, with arsenite being the most toxic, however, both are carcinogenic (Tolins et al., 2014).
Arsenic poisoning is a medical condition that occurs due to elevated levels of arsenic in the body with lots of discomfort in humans and growth malfunction in plants (Davis, 2020). The accumulation of arsenic throughout the food chain causes various disorders, producing potential human health risks and ecological disturbances (Singh et al., 2023). In plants, arsenic is potentially toxic, resulting in retarded growth, poor plant yield, reduced nutrient absorption, plant metabolism disorder, and an impaired ability to fixate molecular nitrogen in leguminous plants (Guala et al., 2010). High exposures in humans can cause skin and lung cancer, pulmonary sickness, skin lesions, cardiovascular disease, diabetes, black foot disease, brain development retardation, hyperkeratosis, and even mortality in young adults (Farzan et al., 2013).
According to the arsenic fact sheet (Tolins et al., 2014; Ng et al., 2003), World Health Organisation’s (WHO’s) provisional guideline value for arsenic levels in drinking water is 10 micrograms per litre (µg/L), a range of 1-40 part per million (ppm) with an average of 5 ppm for soil and 20 ppm for most agricultural soil. As a result of the harm caused to plants and humans by the accumulation of arsenic, arsenic uptake is of global concern due to its tendency to be absorbed by plant roots and transferred to man through the food chain (Li et al., 2016).
1.2 Colocasia esculenta (Cocoyam Plant)
Colocasia esculenta is a tropical plant grown mainly for its edible root and leaves. Its corm is a root commonly known by specific names in different countries (Kalo in Hawaii, Arbi in India, Madumbi in South Africa, cocoyam in Ghana, Koko/Ede in Nigeria, etc.) It belongs to the kingdom Plantae, Family Araceae, Genus Colocasia and has a species name, esculenta (Rashmi et al., 2018; Hill, 1939). The petioles of the leaves (stem) stand straight and tall and can grow to lengths of about a meter. Its leaves are shaped like hearts, are broad and large as shown in Figure 1.
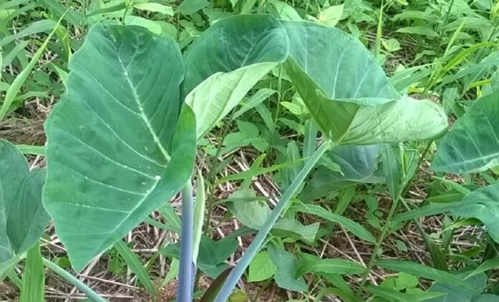
Figure 1: Image of Cocoyam Plant (Colocasia esculenta)
Cocoyam is among the many tropical crops that grow in many countries, serving as a source of carbohydrate and food for more than 400 million people around the world (Brown, 2000). It’s also known as one of the tropical tuber crops such as yam, sweet potato serving as an emergency food crop in many countries such as Ghana, Nigeria, parts of India, Japan, and in tropical countries where they are grown (Owusu-Darko et al., 2014).
This plant is known to contain nutrients that help the body to balance certain dietary requirements and is crucial for a healthy functioning immune system. According to Okpala (2015), cocoyam contains no gluten, more starch, dietary fiber, and higher protein contents than similar tropical root crops. It also contains thiamine, calcium, manganese, vitamin B, vitamin C, vitamin E, magnesium, copper, and riboflavin (Rashmi et al., 2018). Cocoyam leaves are the most useful parts of the plant because they do not only serve as food but also contain plenty of useful vitamins and minerals, and help remove fats and improve major health issues (Ukwu et al., 2022).
The high levels of iron and copper in cocoyam makes important in the prevention of anemia and improved circulation throughout the body, which aid in the production of the red blood cells that transport oxygen throughout the body’s system and cells (Okpala, 2015). The health benefits of cocoyam include; cancer prevention, reduction in symptoms of arthritis, regulation of blood pressure, immune system booster, maintaining digestive health, reducing muscle cramps, boosting vision (sight), preventing diabetes, and, hydrating the skin (Appiyah, 2017).
Roasting, boiling, frying, baking, pounding, milling among others are ways through which cocoyam is prepared for consumption and use. In Ghana, Nigeria, Cameroon, Cote D’Ivoire, and other countries, the corm is cooked and prepared into dishes such as ampesi, akaw, fufu, ‘3tↄ’, ‘fried chips and mpotompoto, among others. The leaves are used to prepare dishes like palava sauce, ‘kontomire’, ‘abomu’, and ‘abunuabunu’. According to Jennings (2009) and Rao et al. (2010), the processing of the root of the plant into flours enhances its economic value by enabling its storage and later use. Snack and food processors and manufacturers can use the plant to produce healthy snacks that are ready to eat or complementary foods that are ready to prepare.
1.3 Phytoremediation
Several ways exist for the cleaning and protection of the environment and green spaces, and one major way is the natural processes of plants as they clean the air. Plants do this by taking up carbon dioxide, one of the major greenhouse gases, and release back into the environment, oxygen. Some plants use their roots to remove potentially harmful pollutants from the soil, collect and store the toxins in their cells, or make them less toxic (Chibuke & Obiora, 2014). This discovery has resulted in the use of several plant species that can help draw out heavy metals like mercury, cadmium, nickel, lead, arsenic, and other toxins from the soil. Phytoremediation thus refers to the use of plants and associated soil microbes to reduce the concentrations or toxic effects of contaminants in the environment (Greipsson, 2011; Ali et al., 2013). Phytoremediation applies to all processes (chemical or physical) that involve the use of plants for soil and groundwater degradation of pollutants. It is the natural ability of plants known as hyperaccumulators to bioaccumulate, degrade, or render harmless contaminants in soils, water, or air (Phillips, 2021). The concept of phytoremediation has been utilized in the removal of heavy metals, and many researchers have employed the use of sunflower (Helianthus annuus), or the Chinese Brake fern (Pteris vittata), and Cocoyam Plant (Colocasia. esculenta) among others for the remediation of arsenic, and most of them have yielded interesting findings and expanded knowledge on arsenic phytoremediation.
1.4 Previous Works
The challenge of arsenic contamination and poisoning across the world had necessitated research on the removal or reduction of the concentrations of arsenic in the environment by the use of Colocasia esculenta, and some of these key researches on this subject are highlighted. Manirul et al. (2015) evaluated arsenic accumulation by C. esculenta using different concentrated arsenic contaminated soil and monitored the experiment from July to October. The plants were grown on the soil for this period, and it was observed that the plant properties and growth decreased significantly as the arsenic contamination level in the soil increased. The study confirmed the accumulation potential of arsenic in the plants’ leaves and its runner. Aksorn and Visoottiviseth (2004), also studied the plants suitable for the removal of arsenic from water. The plants used were Typha spp, Canna spp, Colocasia esculenta, Heliconia psittacorum, and Thalia dealbato J. Fraser. Their experiment lasted for 28 days and a concentration of 1 mg/L arsenate was used. It was discovered that Colocasia esculenta was the best plant for the removal of arsenic from the water, and most of the arsenic accumulates in the plant roots. To identify the ability of some plants to decrease arsenic pollutants from thermal energy wastewater using the phytoremediation process, Yanuwiadi et al. (2013) used Monochoria vaginalis, Salvinia molesta, and Colocasia esculenta for an experiment conducted from June to August. Parts of the plants were digested in Acid and analyzed using Atomic Absorption Spectrophotometer (AAS) after the stipulated period. The results indicated no significant difference in arsenic absorption among the plants. Jomjun et al. (2010) confirmed that Colocasia esculenta can remove arsenic about 68 mg As/m2/day, and was considered as one with the fastest ability to absorb arsenic in wetland environments. All research yielded positive results and have confirmed the possibility of arsenic to be remediated by the cocoyam plant (Colocasia esculenta). However, the literature on arsenic phytoremediation using Colocasia esculenta has failed to analyze the toxicity of the plant after its absorption study. Since this plant is edible and serves as a source of food for millions of people, there is the need to analyze the toxicity of this plant exposed to an arsenic contaminated soil or C. esculenta used for arsenic accumulation (phytoremediation). This study, therefore, investigated the toxicity and edibility of a cocoyam plant (Colocasia esculenta) grown in arsenic-contaminated soil.
2. Resources and Methods Used
2.1 Study Area
Soil sample weighing seven kilograms (7 kg) was collected from a site opposite the main gate of the University of Mines and Technology (UMaT), Tarkwa, a garden where two (2) Colocasia esculenta (cocoyam) plants of relatively equal width, height, and biomass were also uprooted for this study. The site where the soil and plant samples were collected can be located a distance away from the main gate of the university campus, a community known as ‘Kamponase’ in the Tarkwa Nsuaem Municipal area. The site in question is known to be very fertile for plant growth since it is a garden on which pepper and tomatoes are grown for personal use.
2.2 Materials Used
Two (2) mini buckets, 6 liters each, were purchased from the Tarkwa market. The standard arsenic solution, a four thousand (4000) µm screen, an electronic balance, measuring cylinder, concentrated nitric acid (HNO3), concentrated hydrochloric acid (HCl), pH meter, and an electric burner were available at the Minerals Laboratory of UMaT. Shimadzu UV-3600 Plus Ultraviolet Spectrophotometer (UV) was used to read the arsenic concentrations after the analysis.
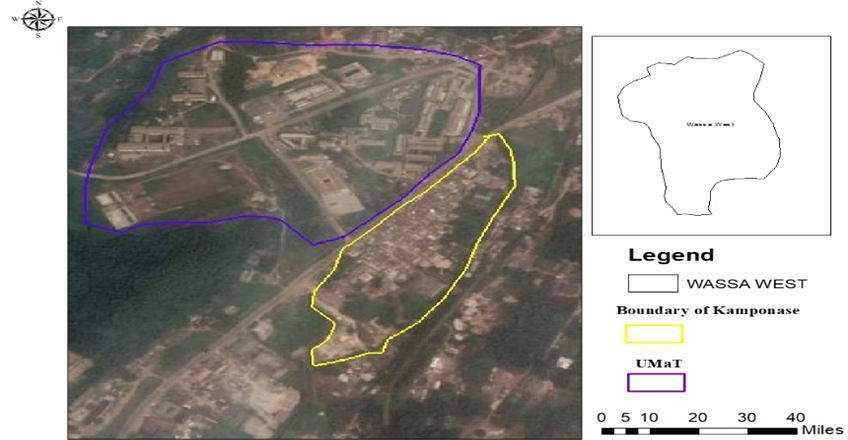
Figure 2: Location of Sampling Site
2.3 Sampling and Preparation
Samples from soil and plant parts (stem, leaves, and root) were taken at the beginning of the project for analysis to ascertain the arsenic levels already present in them before the planting and monitoring period. The soil and plant samples collected were air-dried and sieved using the 4000 µm screen to get rid of rubbers, nails, stones, and other foreign materials and portions were taken for acid digestion from which solution samples were collected for UV analysis.The sample was further sub-divided into two and each of the two planting buckets were filled with the sub-divided soil sample (3000 g) measured using the electronic balance. The two (2) buckets were labeled to represent setups X (main experiment) for 10 ppm arsenic and setup Y (control experiment) for no arsenic addition. A pulp density of 50% solids was prepared for both setups, and 3000 ml of de-ionized water was added to setup Y. For setup X, 10 ppm concentration of arsenic was prepared, out of a 10,000 ppm standard solution, in 3000 ml of de-ionized water. The constituents of both setups were mixed thoroughly to obtain homogeneity.
2.4 Planting
The two Colocasia esculenta plants were planted in setups X and Y respectively. The setups were left in the open environment for four (4) weeks to mimic the behavior of plants in contaminated environments. The plants were allowed to grow, and the growth efficiency was monitored throughout the experiment. Solution samples were taken at the beginning of the experiment, day three, and on weekly basis for the entire period of the experiment. From time to time, de-ionized water was added to all two setups to ensure constant plant growth and to compensate for the water that was lost through plant transpiration and evaporation. Figure 3 shows the setup with fresh cocoyam plants at the inception of the experiment.
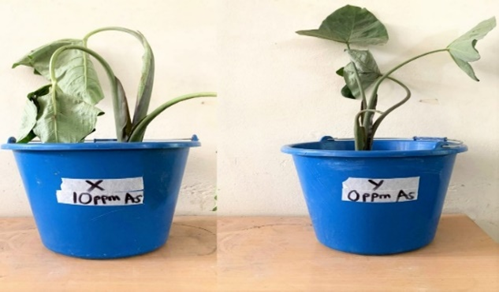
Figure 3: Setup after Planting at 0 hrs
2.5 Sample Analysis
All acid digested samples at the start of the experiment and solution samples taken during the four-(4)-week period, as well as the solid samples digested in acid after the experiment, were analyzed in the Environmental Monitoring Laboratory in UMaT using a SHIMADZU UV-3600 Analyser. The sampling points selected for the Ultraviolet (UV) analysis are presented in Table 1.
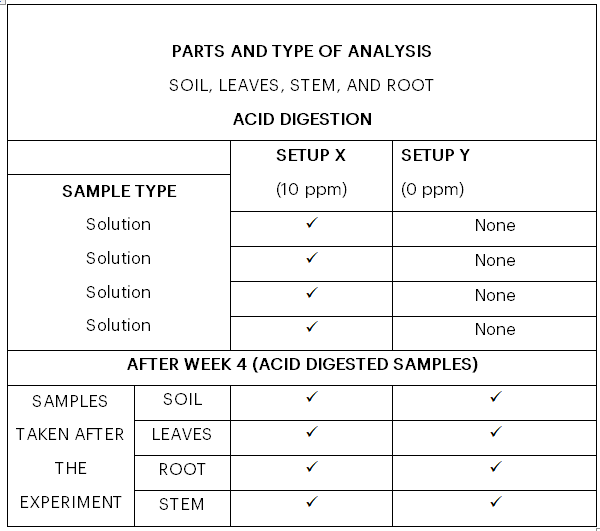
Table 1: Sampling Points for Analysis
3. Results
3.1 Physical Assessment
In the assessment of the plants’ toxicity after exposure to an arsenic-contaminated environment, physical examination aside from the UV analysis was conducted weekly to establish the growth and adaption progress of the plant in its surrounding. Contaminated soil has the potential of transporting toxic materials into the plants in its surrounding through xylems in plants’ roots. These contaminants have the tendency of retarding plant growth and may be observed in the growth process of the plant (inability to grow and reproduce). However for this experiment, within the first week, as depicted in Figure 4, both plants went through adaptation to the new environment, with the original leaves withering and falling off. By the end of the third week (Figure 5), the plants had adapted to their environment with new leaves seen to have sprouted up, and by the end of the fourth week, the plant had grown fully and a positive growth process was observed. The normal growth of the plant across the weeks portrays the ability of a Colocasia esculenta plant to strive and adapt well even in harsh soil conditions. Although naturally, some soil contains arsenic and tend to be a major resting place after its release from the use of pesticides, paint products, and mining activities (Tajer, 2019), the entirety of a Colocasia plant needs to be checked even though it adapts and grows well in an arsenic-contaminated environment. The soil, plant, and arsenic interactions during and after the experiment are further discussed.
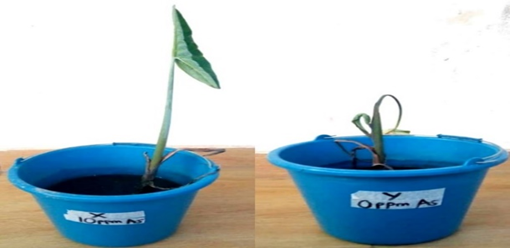
Figure 4: Setup within the First Week
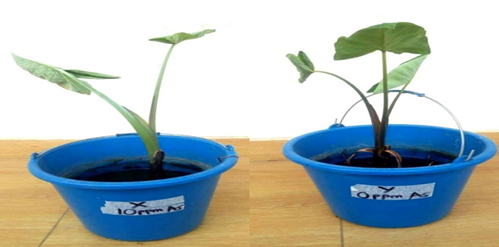
Figure 5: Setup within the Third Week
3.2 Soil–Plant Interaction
According to Fisher (2018), soil plays a crucial role in a plant’s growth because it contains and provides nutrients, water, heat, oxygen, and physical support required for good plant growth. Most plants do not exhibit early signs of heavy metal poisoning although most minerals in the soil dissolve in water and become available to them for uptake at any time. The concentration of arsenic in the soil samples and the plant parts analyzed by acid-digestion before the inception of the experiment showed no significant amount of arsenic present in the soil and the root of the plant, while high levels of 8.5 mg/l and 4.4 mg/l As was recorded in the leaves and the stem of the plant, respectively (Figure 6). These levels may be attributed to naturally existing arsenic levels in the soil as a result of exposure to small-scale mining activities, discarded batteries, wires, and arsenic-containing sources like paints and pesticides. There are no recorded arsenic levels in the soil because, many metals move through the soil to a plant’s root and up its shoot as a result of concentration gradients of nutrients from high to lower concentrations (Fisher, 2018). This mechanism allows some metals to be selectively absorbed up the plant rendering concentrations in the surrounding environment (soil) to be lower than in the plant.
From the experiment, there were changes observed in the plant’s physical growth in terms of adaptation because the plants showed positive growth over the weeks and had adapted to their surrounding environment by the second week. The concentration levels of arsenic in the soil and plant parts also took a drastic change due to the mechanism of translocation as the levels in the soil and plant parts did not remain as that recorded at the inception of the experiment.
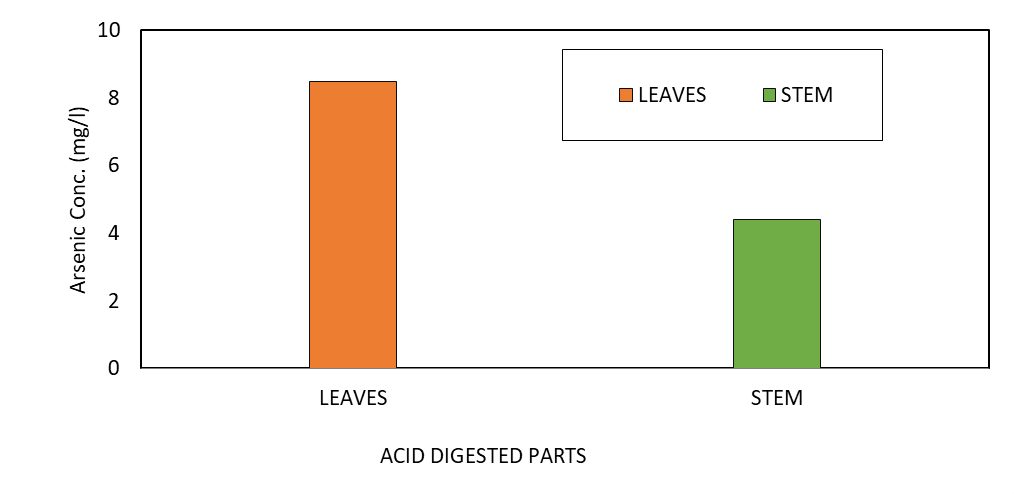
Figure 6: Arsenic Concentration Before Planting
3.3 Bioaccumulation and Translocation of Arsenic
The observed arsenic concentrations in the leaves, stem, and root of the plant were used to measure the Bioaccumulation factor (BAF) and Translocation factor (TF) to determine whether Colocasia esculenta can be categorized as an accumulator or stabilizer (Arnot and Gobas, 2006). The arsenic concentrations in the filtrates after analysis were used to calculate these factors which is a measure of a plant’s ability to accumulate the concentration of a metal in the surrounding soil (Ghosh and Singh, 2005) and its ratio/distribution between the root and the plant shoot respectively. These two factor-indices were used to check the degree of transfer of arsenic from certain parts of the plant to other parts and also the levels of arsenic accumulated in the various parts.
The Translocation Factor (TF) and Bioaccumulation factor (BAF) were calculated using Equations 1 and 2 respectively to explain the great changes noticed in the concentration of arsenic in the soil and the plant parts. According to Wang et al. (2019) plants with a BAF >>1 are considered as accumulators and TF >>1 indicates a plants’ high translocation capacity and its ideality for phytoabsorption whereas TF <<1 is ideal for phytostabilization (Yoon et al., 2006).
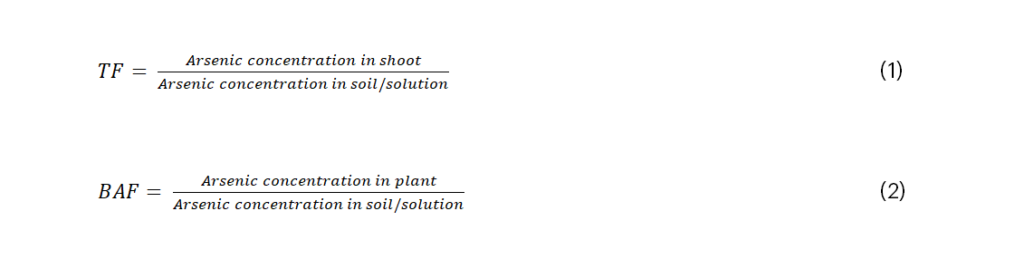
The calculated BAF across setup X indicates that Colocasia esculenta is a potential hyperaccumulator of arsenic demonstrated with a high BAF of 1.43 as shown in Table 2. The TF as shown in Table 2 indicates that a significant amount of arsenic was translocated to the shoot (leaves and stem) from the root and soil.
Since higher TF values are expected in plants for better ability to transport metals from roots to shoots, hence its importance in phytoremediation (Wang et al., 2019), Colocasia esculenta can be considered for this purpose. The translocation of arsenic from the stem to the leaves was very minimal, and this explains why most of the arsenic was concentrated more in the stem than the leaves. The lower TF value of 0.26 calculated for translocation from the stem to the leaves indicates this plant is a good phytostabilizer and can be considered for the process of phytomining. However, these uptake factor values calculated explain the qualitative aspect of accumulation and translocation rather than the quantitative one.
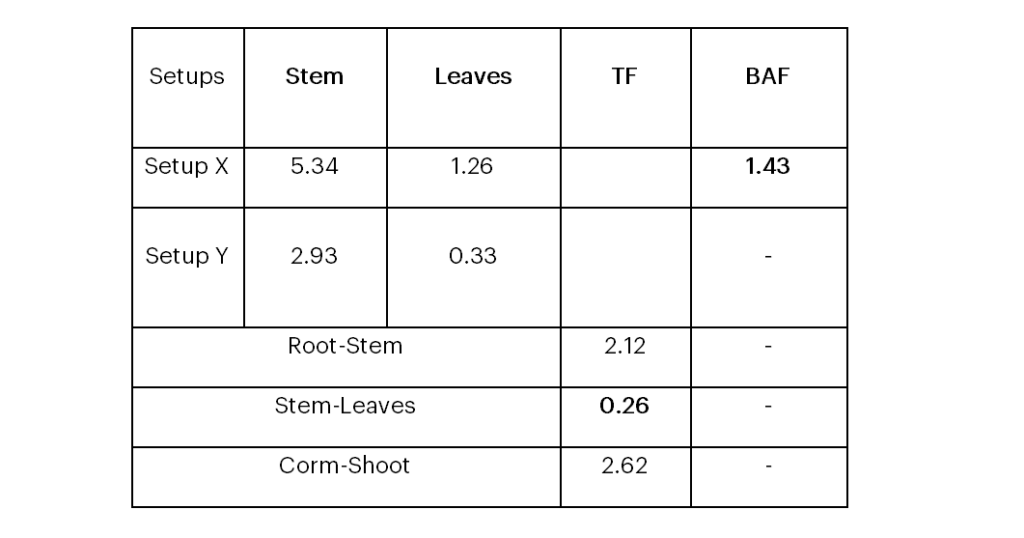
Table 2: Bioaccumulation and Translocation Factors
3.4 Arsenic Concentration in Solution
The absorbance against wavelength graph shown in Figure 7 depicts the trend of arsenic concentration in the solution samples collected on the first and third day as well as the first and second weeks. This graph is a plot of arsenic absorbance in the samples collected over the weeks against wavelength (nm) with values comparable to an arsenic known standard. This analysis was conducted for the main experiment (Setup X) to monitor arsenic left in the planting solution over time. It can be inferred from the comparison that there was a negative correlation with increasing days as the level of arsenic in solution decreased over days and ended up with almost nothing in solution by the second week. The 10 ppm arsenic concentration in the soil at the inception of the experiment was shown to have reduced across the days and almost showed less arsenic absorbance by the later weeks indicating the potential of arsenic uptake from the soil and up the Colocasia esculenta plant over the observed period.
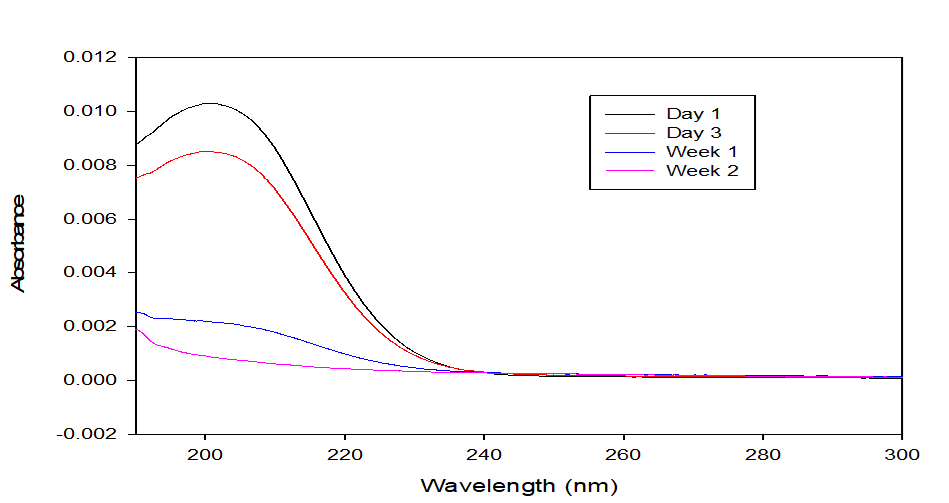
Figure 7: Arsenic Absorbance against Wavelength (nm)
3.5 Arsenic Levels in Soil and Plant Parts after Experiment
Setup Y (Control) showed lower levels of arsenic as compared to the main experiment with the highest concentration recorded in the soil (Figure 8) for setup X. The soil and root which initially had no amount of arsenic at the inception of the experiment had increased arsenic concentrations whilst the leaves which had the highest concentration before the experiment gave off most of their arsenic resulting in its arsenic reduction. There were changes in arsenic levels in both experiments as compared to the initial levels already present in the plant. Some parts lost arsenic while others reduced, and this can be explained based on the various exchange mechanisms that take place in plants. Arsenic translocation efficiency from the roots to the shoots affects its tolerance in plants and determines the ability of the Colocasia esculenta to accumulate arsenic (Li et al., 2016). However, most edible parts of a plant as a result of long-distance translocation of arsenic, result in accumulation in its edible tissues, posing serious risks to human health. In this study, the long distance transport mechanism resulted in the tissue transfer of arsenic from the leaves and roots to other parts concentrating more in the plants’ stem.
Comparing the initial and final concentrations of arsenic in the various plant parts across the setups, the levels of arsenic in the control experiment (setup Y) existed from arsenic already present in the plant’s leaves and stem before the experiment. In Setup X, the highest arsenic level was recorded in the soil after the experiment although no significant amount was shown in the solution samples taken by the last week indicating the potential of arsenic to stay in soil with fewer traces in solution for plant uptake as a result of its change of state. Increased levels of arsenic in the experimental setup by comparison with the control indicates the uptake and translocation potential of arsenic in the soil through different mechanisms up a Colocasia esculenta plant into its parts.
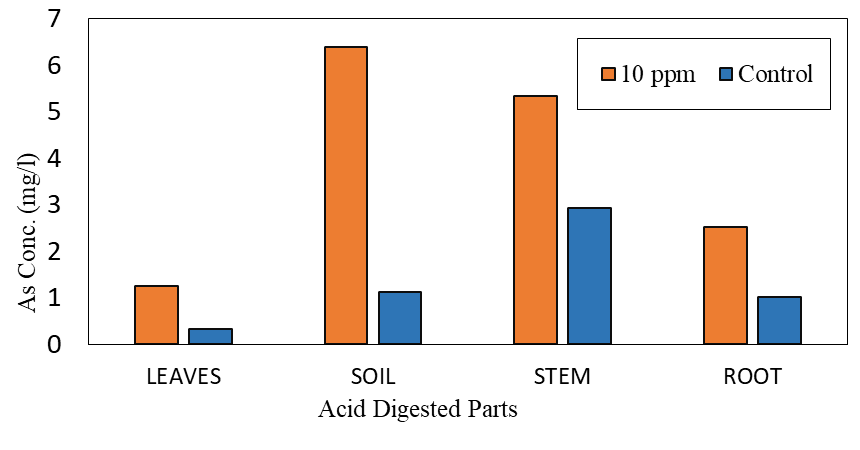
Figure 8: Arsenic Concentration after the Experiment
There was a major change in concentration in the plant parts, and this was due to the translocation of different levels of arsenic across the parts as explained with the arsenic levels in solution over time. The loss of arsenic found from the deduction of arsenic in the plant before and after the experiment is due to phytovolatilization and transpiration which is the loss of water and some chemical compounds from a
plant into the environment through the leaves of the plant. In this mechanism contaminants present in the water that is taken up by the plant, pass through the plant and/or are modified by the plant, and are released to the atmosphere as vapor. Comparatively, Figure 9 shows the arsenic distribution across the plant parts expressed in percentage at the end of the fourth week. It can be seen that majority of the metal was accumulated in the stem followed by the root and the leaves with percentage values of 58%, 28%, and 14% respectively.
Alternatively, Figure 10 shows the graph of absorbance against wavelength based on the UV-VIS analysis for Setups Y and X, respectively, after the experiment depicting the trend of arsenic present in the plant part. Figures 10 (a) and (b) represent the comparison made between the control (Setup Y) and the main experiment (Setup X). The peaks show the level of arsenic absorbance recorded in the solution samples analyzed. Arsenic absorbance trend in the control experiment was of the format Stem > Root > Soil > Leaves and was also of the format Soil > Stem > Root > Leaves for the main experiment. This varying trend in arsenic uptake in the two experiments shows that there is no definite order for arsenic uptake and storage in a C. esculenta plant, however, arsenic will be absorbed up through the plants’ root and translocated to its shoot over some time.
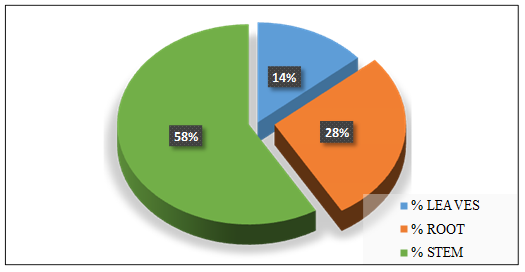
Figure 9: Arsenic in Plant Parts after Experiment (Setup X)
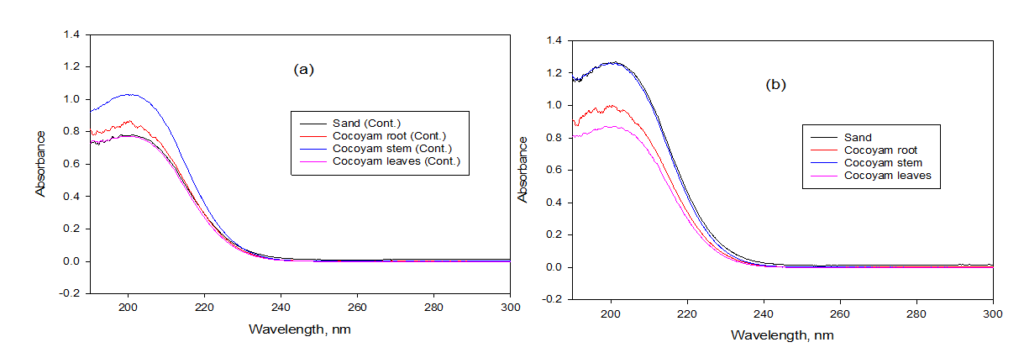
Figure 10: Arsenic Absorbance in (a) Control and (b) Main Experimental Setup
4. Plant Edibility
As a result of the carcinogenic effect of arsenic in humans the World Health Organisation’s (WHO) permissible level of arsenic in foods and drinking water is 0.001 ppm or 10 µg/L (Rahman et al., 2010), and a range of 1-40 ppm with an average of 5 ppm arsenic recommended in most soil (Ng et al., 2003), for agricultural purposes. According to arsenic facts sheets, water above this level is not safe, hence not recommended for domestic use, and all these standards are approved by the Food and Drugs Authority (FDA) as well as the Environmental Protection Agency (EPA). By comparison of the arsenic levels in the C. esculenta plant after the experiment as shown in Table 4 with these recommended standards, the arsenic levels observed are higher than expected. The initial and final arsenic levels in the C. esculenta plant in this study indicate the potential of arsenic to be accumulated and translocated in its tissues somehow over some time and this is a great threat to plant and animal life. Although most of the arsenic was accumulated in the stem which is not edible, the consumption of this plant (leaves and root) is .questionable, taking into consideration arsenic poisoning through the food chain since knowledge of the arsenic state (organic/inorganic) contained is not known.
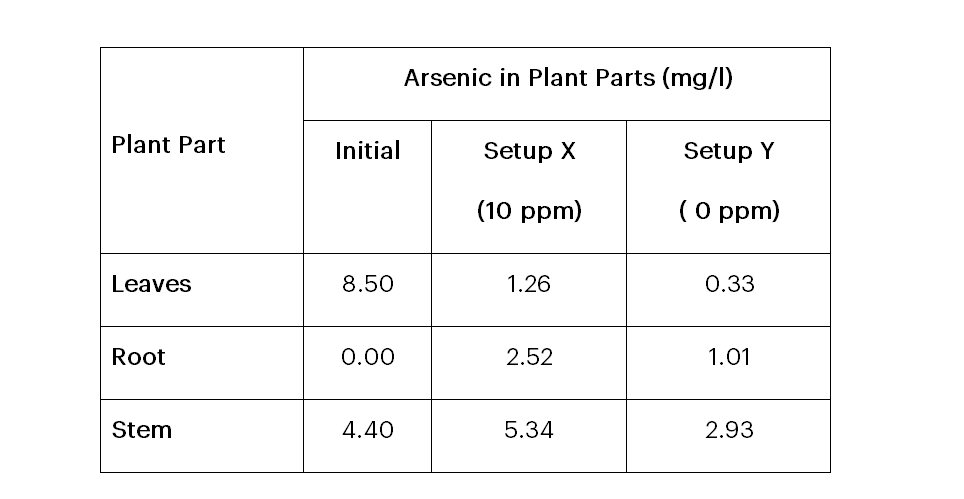
Table 4: Arsenic in Plant Parts
5. Conclusion
This study has shown Colocasia esculenta is a potential plant for arsenic uptake from contaminated soil and water. The evident indicators of arsenic uptake analyzed in this experimental work showed high Translocation Factor (TF) and Bioaccumulation Factor (BAF) values of greater than 1. The BAF value greater than one (>1) establishes that Colocasia esculenta is an accumulator or hyperaccumulator. The higher TF value calculated for the soil to the shoot indicates the plant’s high translocation factor, hence ideal for phytoabsorption.
The lower TF value of 0.26 for the stem to the leaves indicates that this plant is a good phytostabilizer and can be considered for the process of phytomining. UV analysis conducted on the weekly samples taken showed a declining trend in arsenic concentration levels confirming the uptake process. With a focus on the plant, the highest arsenic level was recorded in the stem at 58% followed by the root at 28% and the leaves at 14%. By comparison with the World Health Standard, it is not advisable to consume cocoyam planted in an arsenic-contaminated area and, hence, its edibility is questionable. Based on the results obtained, care is to be taken as to where Colocasia esculenta plants are planted, its source of irrigation, and the nature of its environment when it is grown as a source of food. Laboratory experiments should be conducted to know the state of arsenic (organic or inorganic) in the Colocasia esculenta plant after arsenic uptake to know the level of its toxicity before it is generally used for phytoremediation processes. Further work could be done to establish the actual mechanism of arsenic uptake by the cells of the plant and the metal inventory in the plant cells. Field studies can also be performed for an extended number of months to ascertain the arsenic uptake potential and trend of the plant over an extended period.
Author(s) Summary
Acknowledgments
The authors acknowledge and express sincere thanks to the staff of the Minerals Engineering Department of the University of Mines and Technology (UMaT), Tarkwa, and most especially to Dr. Clement Owusu, Dr. Eric Agorhom, and Theophilus Wereko for their technical assistance and support.
Competing Interest
The authors declare that they have no competing interests.
References
Ali, H., Khan, E., & Sajad, M. A. (2013). Phytoremediation of Heavy Metals—Concepts and Applications. Chemosphere, 91(7), 869-881. [Google Scholar] [CrossRef]
Ali, H., Khan, E., & Ilahi, I. (2019). Environmental Chemistry and Ecotoxicology of Hazardous Heavy Metals: Environmental Persistence, Toxicity, and Bioaccumulation. Journal of Chemistry, 2019. [Google Scholar] [CrossRef]
Aksorn, E., & Visoottiviseth, P. (2004). Selection of Suitable Emergent Plants for Removal of Arsenic from Arsenic Contaminated Water. Science Asia, 30(2), 105-113. [Google Scholar] [CrossRef]
Appiyah, L. (2017, February). On the Surprising Nutrition Facts about Cocoyam Roots or Taro (Colocasia Esculenta). Nobowa. [CrossRef]
Arif, N., Yadav, V., Singh, S., Singh, S., Ahmad, P., Mishra, R. K. & Chauhan, D. K. (2016). Influence of High and Low Levels of Plant-Beneficial Heavy Metal Ions on Plant Growth and Development. Frontiers in Environmental Science, 4(69). [Google Scholar] [CrossRef]
Arnot, A., & Gobas, A. (2006). A Review of Bioconcentration Factor (BCF) and Bioaccumulation Factor (BAF) Assessments for Organic Chemicals in Aquatic Organisms. Environmental Reviews, 14(4), 257-297.[Google Scholar] [CrossRef]
Brown, D. (2000). Aroids, Plants of the Arum Family. Oregon: Timber Press.
Chibuike, G. & Obiora, C. (2014). Heavy Metal Polluted Soils: Effect on Plants and Bioremediation Methods. Applied and Environmental Soil Science, 2014. [Google Scholar] [CrossRef]
Davis, P. (2020, July). Arsenic poisoning. Retrieved August 28, 2022 from [Website]
Farzan, S., Karagas, R. & Chen, Y. (2013). In Utero and Early Life Arsenic Exposure in Relation to Long-term Health and Disease. Toxicology and Applied Pharmacology, 272(2), 384-390. [Google Scholar] [CrossRef]
Fisher M. (2018). Environmental Biology. New York: McGraw-Hill Primis Custom Publishing.
Ghosh, M., & Singh, P. (2005). A Comparative Study of Cadmium Phytoextraction by Accumulator and Weed Species. Environmental Pollution, 133(2), 365-371. [Google Scholar] [CrossRef]
Greipsson, S. (2011). Phytoremediation. Nature Education Knowledge, 3(10), 1-7
Guala, S. D., Vega, F. A., & Covelo, E. F. (2010). The Dynamics of Heavy Metals in Plant–Soil Interactions. Ecological Modelling, 221(8), 1148-1152. [Google Scholar] [CrossRef]
Hill F. A (1939). The Nomenclature of the Taro and its Varieties. Botanical Museum Leaflets, 7(7), 113-118. [Google Scholar] [CrossRef]
Jang, C., Somanna, Y. & Kim, H. (2016). Source, Distribution, Toxicity and Remediation of Arsenic in the Environment–A Review. International Journal of Applied Environmental Sciences, 11(2), 559-581. [Google Scholar]
Jennings, D. (2009). Tropical Root and Tuber Crops: Cassava, Sweet Potato, Yams and Aroids. Experimental Agriculture, 45(3), 382. [Google Scholar] [CrossRef]
Jomjun, N., Siripen, T., Maliwan, S., Jintapat, N., Prasak, T., Somporn, C., & Petch, P. (2010). Phytoremediation of Arsenic in Submerged Soil by Wetland Plants. International Journal of Phytoremediation, 13(1), 35-46. [Google Scholar] [CrossRef]
Li, N., Wang, J. & Song, W. (2016). Arsenic Uptake and Translocation in Plants. Journal of Plant and Cell Physiology, 57(1), 4-13. [Google Scholar] [CrossRef]
Manirul, I., Mayeda, S., & Uddin, A. (2015). Evaluation of Arsenic Accumulation by Colocasia Esculenta (Kochu) from Soil. Journal of Soil and Nature, 8(2), 1-4.
Mojiri, A., Aziz, H. A., Zahed, M. A., Aziz, S. & Selamat, M. (2013). Phytoremediation of Heavy Metals from Urban Waste Leachate by Southern Cattail (Typha domingensis). International Journal of Scientific Research in Environmental Sciences, 1(4), 63-70. [Google Scholar] [CrossRef]
Ng, J. C., Wang, J., & Shraim, A. (2003). A Global Health Problem Caused by Arsenic from Natural Sources. Chemosphere, 52(9), 1353-1359. [Google Scholar] [CrossRef]
Okpala, B. (2015). On the Benefits of Cocoyam (Taro/Colocasia Esculenta). Retrieved August 28, 2022 from [Website]
Rashmi, D. R., Raghu, N., Gopenath, T. S., Palanisamy, P., Bakthavatchalam, P., Karthikeyan, M., & Basalingappa, K. (2018). Taro (Colocasia esculenta): An Overview. Journal of Medicinal Plants Studies, 6(4), 156-161. [Google Scholar]
Owusu-Darko, P. G., Paterson, A., & Omenyo, E. L. (2014). Cocoyam (corms and cormels)—An Underexploited Food and Feed Resource. Journal of Agricultural Chemistry and Environment, 3(01), 22-29. [Google Scholar] [CrossRef]
Phillips, T. (2021). On the 6 Types of Phytoremediation. Retrieved September 12, 2022, from [Website]
Rahman, S., Abdul Mazid Miah, M., Khaled, M., Islam, A. & Panaullah, M. (2010). Arsenic Concentrations in Groundwater, Soils, and Irrigated Rice in Southwestern Bangladesh. Communications in Soil Science and Plant Analysis, 41(16), 1889-1895. [Google Scholar] [CrossRef]
Rao, V., Matthews, P., Eyzaguirre, P. & Hunter, D. (2010). The Global Diversity of Taro: Ethnobotany and Conservation. Rome, Italy: Biodiversity International. [Google Scholar]
Singh, J. & Kalamdhad, S. (2011). Effects of Heavy Metals on Soil, Plants, Human Health and Aquatic Life. International Journal of Research in Chemistry and Environment, 1(2), 15-21. [Google Scholar]
Singh, S., Yadav, R., Sharma, S., & Singh, N. (2023). Arsenic Contamination in the Food Chain: A Threat to Food Security and Human Health. Journal of Applied Biology & Biotechnology X(XX), 1-10. [Google Scholar] [CrossRef]
Tajer, A. (2019). Should You Worry that Your Soil is Contaminated? Greenway Biotech Inc. Retrieved August 12, 2022, from [Website]
Tolins, M., Ruchirawat, M. & Landrigan, P. (2014). The Developmental Neurotoxicity of Arsenic: Cognitive and Behavioral Consequences of Early Life Exposure. Annals of Global Health, 80(4), 303-314. [Google Scholar] [CrossRef]
Ukwu, P., Yahaya, A., Okere, C., Aladi, N., Odoemenam, U., Obikaonu, H. O. & Okoli, C. (2022). The Production, Uses, Nutritional and Anti-nutritional Characteristics of Cocoyam as a Potential Feed Ingredient in the Tropics: A Review. Nigerian Journal of Animal Science, 24(3), 91-111. [Google Scholar]
Wang, Z., Liu, X. & Qin, H. (2019). Bioconcentration and Translocation of Heavy Metals in the Soil-plants System in Machangqing Copper Mine, Yunnan Province, China. Journal of Geochemical Exploration, 200, 159-166. [Google Scholar] [CrossRef]
Yanuwiadi, B. & Polli, B. (2013). Phytoremediation of Arsenic from Geothermal Power Plant Waste Water Using Mono Vaginalis, Salvinia Molesta and Colocasia Esculenta. International Journal of Bioscience (IJB), 3(6), 104-111. [Google Scholar] [CrossRef]
Yoon, J., Cao, Z. & Lena, M. (2006). Accumulation of Pb, Cu, and Zn in Native Plants Growing on a Contaminated Florida Site. Science of the Total Environment, 368(2-3), 456-464. [Google Scholar] [CrossRef]
About this Article
Cite this Article
APA
Amosah, M. E., Asare D. A, Anderson, E., & Ofori-Sarpong G. (2023). Edibility of Cocoyam Plant (Colocasia esculenta) in an Arsenic-Contaminated Soil. SustainE. 1(1), 62-80. https://doi.org/10.55366/suse.v1i1.5
Chicago
Amosah, Margaret Ekua, Deborah Asarebea Asare, Eugenia Anderson , and Grace Ofori-Sarpong. “Edibility of Cocoyam Plant (Colocasia Esculenta) in an Arsenic-Contaminated Soil.” SustainE 1, no. 1 (May 1, 2023): 62–80. https://doi.org/10.55366/suse.v1i1.5
Received
14 November 2022
Accepted
20 April 2023
Published
1 May 2023
Corresponding Author Email: margaretekuaamosah@gmail.com
Disclaimer: The opinions and statements expressed in this article are the authors’ sole responsibility and do not necessarily reflect the viewpoints of their affiliated organizations, the publisher, the hosted journal, the editors, or the reviewers. Furthermore, any product evaluated in this article or claims made by its manufacturer are not guaranteed or endorsed by the publisher.
Distributed under Creative Commons CC-BY 4.0
Share this article
Use the buttons below to share the article on desired platforms.