Popoola O. A.;1 Agarry O. O.;2 & Orukotan A. A.3
1Genetics, Genomics and Bioinformatics Department, National Biotechnology Research Development Agency (NBRDA), Abuja, Nigeria
2Department of Microbiology, University of Abuja, Abuja, Nigeria
*Corresponding Author Email:atinuke.solina@gmail.com
Abstract
Antimicrobial peptides (AMPs) are gaining recognition as biological preservatives due to their effectiveness against foodborne pathogens and spoilage microorganisms. While widely studied in dairy products, their application in non-dairy food systems remains underexplored. AMPs present advantages over chemical preservatives, such as reduced chemical buildup and targeted microbial action, making them safer and more environmentally friendly for food preservation. This review evaluates the potential of AMPs in non-dairy matrices, including soy milk, plant-based beverages (e.g., zobo), fruits (e.g., pears, mangoes) and vegetables. The mechanisms of action, effectiveness and adaptability of AMPs in fermented and minimally processed foods are examined. Recent advancements in AMP research, such as encapsulation with biocompatible materials (e.g., alginate microparticles) and genetic engineering, have improved their stability, thermostability and resistance to extreme pH conditions, thereby enhancing their applicability in food systems. Despite these advancements, challenges remain, including costly and complex AMP production methods that hinder scalability and the need for comprehensive toxicity evaluations to ensure consumer safety. Biosynthesis and recombinant techniques, though effective, require optimisation for large-scale use. This review synthesises findings from interdisciplinary studies, highlighting AMPs’ potential to extend shelf life, enhance food safety and support sustainable preservation practices. Special attention is given to their relevance in the Nigerian and global food industries. Practical applications and strategies to address current challenges—such as scalability, stability and regulatory compliance—are proposed, paving the way for AMPs to revolutionise non-dairy food preservation. This work aligns with the growing demand for natural, effective and eco-friendly alternatives to conventional preservatives, providing a framework for their widespread adoption in the food industry.
Keywords: Antimicrobial peptides, Non-dairy matrices, Biological preservation, Food safety innovation, Plant-based foods, Sustainability.
1. Introduction
Recently, a rising global demand for safer, healthier and more sustainable food preservation methods has been driven by shifting consumer preferences for natural ingredients, stricter regulatory standards and growing environmental concerns over chemical preservatives. Traditional methods—such as chemical preservatives, refrigeration and pasteurisation—are facing growing scrutiny due to their potential negative impacts, including toxicity risks, depletion of natural resources and degradation of food quality and nutritional value (Obahiagbon and Ogwu, 2024). The rise of plant-based diets, alongside the growing market for non-dairy alternatives, has further fuelled the need for innovative solutions to ensure food safety and prolong shelf life without compromising nutritional value or sensory properties (Sabaté and Soret, 2014). Within this context, antimicrobial peptides (AMPs), which are short chains of amino acids with antimicrobial properties, have emerged as promising natural biological preservatives, offering an alternative to synthetic preservatives (Sharma et al., 2024).
AMPs are naturally occurring substances with wide-ranging antimicrobial properties, effective against various pathogens such as Escherichia coli, Staphylococcus aureus, Candida albicans and enveloped viruses like influenza. These peptides, found in many organisms such as bacteria, fungi, plants and animals, have gained attention in food science for their potential to protect food products from microbial spoilage and contamination (Erdem Büyükkiraz and Mesmen, 2022). Although much of the research on AMPs has focused on their application in dairy products, the application of AMPs in non-dairy matrices remains largely unexplored. Early studies on AMPs in food preservation focused on dairy products, where their ability to inhibit microbial growth and enhance shelf life was recognised (Singh et al., 2023a; Liu et al., 2021). Non-dairy products—including plant-based beverages, fermented foods and minimally processed products—are highly susceptible to microbial contamination by organisms such as Salmonella, Escherichia coli, Lactobacillus, Saccharomyces cerevisiae, Penicillium species and Campylobacter jejuni, which can compromise product safety, quality and shelf life, making biological preservation a crucial area of development (Mishra et al., 2024).
AMPs possess antimicrobial properties primarily through mechanisms such as membrane disruption, enzyme inhibition and interference with cellular processes in microbes (Luo and Song, 2021; Moravej et al., 2018; Guilhelmelli et al., 2013). These properties make AMPs an attractive alternative to chemical preservatives—such as sodium benzoate, potassium sorbate and sulphites—which are increasingly being rejected due to concerns about health risks and consumer preferences for natural ingredients (Harshitha et al., 2024; Novais et al., 2022).
Recent advancements in biotechnology, including innovations in peptide synthesis, purification techniques and bioengineering, have significantly expanded the potential applications of AMPs (Avinash et al., 2024; Kumar et al., 2024). Despite these advancements, several challenges must be addressed before AMPs can achieve widespread adoption in commercial food preservation. Key issues include ensuring AMP stability in diverse food matrices, overcoming interactions between AMPs and food components, addressing regulatory hurdles for AMP use in food, developing scalable production methods and conducting thorough toxicity evaluations to ensure safe consumption (Patil et al., 2022). Peptide stability refers to how well AMPs maintain their structure and function over time, ensuring they remain active and effective in preserving food. If AMPs break down or lose their activity too quickly, they cannot effectively protect food from spoilage or pathogens. Interactions with food components—such as fats, sugars and proteins—can sometimes reduce AMP effectiveness or even make them less stable. Additionally, the specificity of AMPs for non-dairy matrices and their interactions with the complex compositions of plant-based foods (e.g., varying protein structures in soy or the high fibre content in almond milk) necessitate further investigation to understand their mechanisms of action and optimise their efficacy (Devi et al., 2023; Hidalgo-Fuentes et al., 2024).
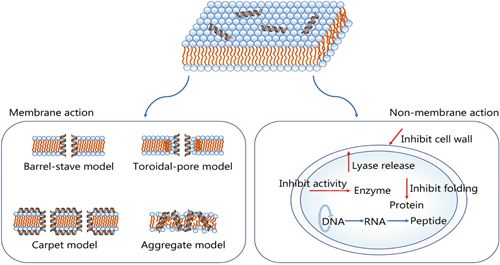
Figure 1: Mechanism of Action of Antimicrobial Peptides (AMPs) (Zhang et al., 2021b)
Foundational studies on AMPs have focused on dairy matrices, where the interactions between peptides and milk proteins have been extensively studied; however, research in non-dairy matrices remains limited (Nongonierma and FitzGerald, 2016). In non-dairy matrices, research is more limited due to the increased complexity of plant-based food systems, which often contain diverse protein structures, high fibre content and varying levels of fats and sugars. These factors can affect the stability and efficacy of AMPs. In addition, the lack of standardised methods for evaluating AMPs in these complex matrices, along with limited funding and resources for research outside the dairy industry, has hindered progress in exploring their potential in non-dairy food products. Early work in this area demonstrated the antimicrobial effectiveness of AMPs in plant-based foods such as soy milk, fruit juices and fermented plant-based beverages, suggesting their potential as biological preservatives in these systems (Horlacher et al., 2023; Shwaiki et al., 2022; Kapetanakou et al., 2021). For example, AMPs have been used in soy milk to target pathogens such as Staphylococcus aureus ATCC 25923, Escherichia coli ATCC 25922 and Listeria monocytogenes. Studies have shown a significant reduction in microbial load over 21 days when soy milk is stored under refrigerated conditions (4°C) (Ma et al., 2020; Xiang et al., 2017). In another study by Iglesias et al. (2018), Lactobacillus rhamnosus GG was applied to fruits such as pears and mangoes to target Listeria monocytogenes. This treatment resulted in a reduction of microbial load over 9 days when the fruits were stored under refrigerated conditions (4°C). Similarly, Sani et al. (2024) applied the antimicrobial peptide P4—derived from germinated seeds of Pisum sativum—to zobo (a plant-based beverage) to target Bacillus cereus, with results showing a reduction in microbial load over 3 days under refrigerated conditions (4°C). These studies highlight the ability of AMPs to prevent microbial spoilage and enhance food safety, although challenges such as peptide instability in non-dairy matrices and potential interactions with food components (e.g., proteins, fats and carbohydrates) have been noted (Rasika et al., 2021; Mäkinen et al., 2016).
Recent advancements have focused on the optimisation of AMP production through biotechnological methods such as recombinant DNA technology and peptide synthesis. For instance, recombinant DNA technology has been employed to insert genes encoding AMPs into host organisms like Escherichia coli, allowing for large-scale production of these peptides. This method has proven effective in increasing yield and reducing production costs, making AMPs more viable for use in food preservation (Sampaio de Oliveira et al., 2020; Wibowo and Zhao, 2019). Researchers have also explored the synergistic effects of AMPs with other preservation techniques, including non-thermal techniques such as high-pressure processing, mild heat treatment and fermentation (Karbowiak et al., 2023; Andaluz-Mejía et al., 2022). In addition, the incorporation of AMPs into edible coatings and packaging materials has shown promise, as these technologies can extend shelf life while maintaining the nutritional and sensory qualities of non-dairy products (Pandey et al., 2022; Liu et al., 2021; Tkaczewska, 2020; Santos et al., 2018). Despite these advancements, challenges remain in scaling up production, ensuring AMP stability in complex food matrices and overcoming regulatory barriers that prevent widespread adoption (Davoudi et al., 2024; Mihooliya and Kumari, 2024).
A major gap in the current literature is the limited understanding of how AMPs interact with the diverse array of non-dairy food components. For example, the complex polysaccharides found in plant-based beverages such as soy or almond milk may interfere with AMP activity and stability, requiring further investigation to optimise their efficacy in these matrices. Unlike dairy matrices, which are relatively homogenous, non-dairy foods vary significantly in composition, which may affect the activity and stability of AMPs (Rivero-Pino et al., 2023; McClements et al., 2021). In addition, while some studies have reported the antimicrobial efficacy of AMPs in non-dairy products, few have addressed their potential for long-term shelf-life extension, particularly in the face of microbial resistance—a growing concern in the food industry (Singh et al., 2023a). Furthermore, the scalability of AMP production and the economic feasibility of using these peptides in commercial food preservation remain underexplored.
This review seeks to bridge existing knowledge gaps by providing a comprehensive analysis of antimicrobial peptides (AMPs) in non-dairy food matrices. It evaluates the antimicrobial effectiveness of AMPs across various non-dairy matrices, with a focus on their stability, activity and interactions with food components. In addition, the review identifies key challenges in their application, including stability under storage conditions, potential toxicity and the regulatory frameworks governing their use in food preservation. Recent technological advancements that enhance the production, purification and application of AMPs in non-dairy products are also examined. Finally, the review proposes future research directions, emphasising the development of cost-effective production methods and the exploration of AMP synergy with other preservation techniques to address current knowledge gaps and advance their commercial viability.
By synthesising existing literature, this review aims to provide a comprehensive understanding of AMPs as a promising solution for biological preservation in non-dairy food systems. It explores both the prospects and challenges associated with incorporating AMPs into these systems, with a specific focus on their ability to extend shelf life, enhance food safety and promote sustainable preservation practices. Through this synthesis, the review highlights the current potential of AMPs while addressing critical knowledge gaps, including issues related to their stability, interaction with non-dairy food matrices and scalability for commercial application. Furthermore, the review outlines key areas for future research that could drive the integration of AMPs into biological preservation strategies, offering practical solutions for overcoming current challenges. By positioning AMPs as a central component in the evolving landscape of food safety, nutrition and sustainability, this review underscores their potential to make a significant impact on both developed and emerging food markets globally.
2.Material and Methods
2.1 Method
This review paper was conducted through a systematic analysis of the current literature on antimicrobial peptides (AMPs) and their application in non-dairy food systems for biological preservation. A comprehensive search was performed across several scientific databases, including PubMed, Scopus and Google Scholar, focusing on studies published between 2000 and 2024. This timeframe was chosen because of the significant advancements in AMP research during this period, which has seen substantial developments in the identification, synthesis and application of AMPs, particularly in food preservation. It also aligns with the increasing interest in natural preservatives and the growing demand for safer, more sustainable food preservation methods in response to consumer and regulatory pressures. Recent breakthroughs in biotechnological methods, such as recombinant DNA technology and peptide synthesis, have contributed to the expansion of AMP research, making this period particularly relevant for examining progress in the field.
The inclusion criteria for selecting articles were based on language, publication type, subject focus and methodology. Only studies published in English were included to ensure consistency and accessibility. Peer-reviewed journal articles, reviews, conference papers and relevant grey literature were considered, with an emphasis on research directly related to the use of AMPs in non-dairy matrices—such as plant-based beverages, fermented foods and minimally processed plant-based products. Grey literature, including industry research reports, governmental publications and technical documents, was also included as it can offer valuable insights not captured in traditional peer-reviewed journals. Such literature often provides real-world applications, preliminary findings and industry-driven innovations that may not yet be fully published or validated in academic journals. The inclusion of both peer-reviewed and grey literature ensures a well-rounded exploration of the current state of AMP research and its potential applications in non-dairy food systems, offering a broader view that complements academic findings with practical and evolving insights.
Studies that examined the antimicrobial efficacy, stability and practical applications of AMPs in these food systems were prioritised, while research outside the specified timeframe or unrelated to AMPs in non-dairy foods was excluded. Global studies were included, with particular focus on regions where non-dairy food systems are rapidly expanding, such as North America, Europe and emerging markets in Asia and Africa. The review also prioritised experimental studies, systematic reviews and theoretical papers that provided valuable insights into the mechanisms and potential challenges associated with AMP-based biological preservation in non-dairy foods.
Once the relevant studies were identified, key data were extracted systematically. This included the types of AMPs studied, their sources (e.g., bacteria, plants or animals), their antimicrobial effectiveness against spoilage organisms and pathogens in non-dairy matrices, and their stability in these systems. The reviewed studies reveal several key trends in the types and sources of AMPs utilised for non-dairy food preservation. A prominent trend is the increasing use of bacteriocins—a group of AMPs primarily derived from bacteria—which have been found to exhibit strong antimicrobial activity against a range of spoilage organisms and pathogens in non-dairy matrices. These include Lactobacillus spp.-derived AMPs, such as nisin, which have shown effective inhibition against Listeria monocytogenes and Escherichia coli in plant-based beverages and fermented foods. Additionally, plant-derived AMPs—for example, those from Pisum sativum (pea)—have gained attention for their potential in food preservation, offering advantages such as lower toxicity and greater biocompatibility with plant-based food systems. Animal-derived AMPs, such as cecropin P1, have also been used in various studies, demonstrating effectiveness in controlling microbial load in fruit juices and other plant-based products.
There is a growing trend in the incorporation of natural AMPs from diverse sources to address microbial contamination in plant-based foods. The studies suggest that the antimicrobial effectiveness of these peptides is often tied to their structural properties. Additionally, information on the application of AMPs for biological preservation, as well as the challenges and limitations reported in the literature, was gathered. Specific challenges related to peptide stability, production costs and regulatory barriers were emphasised, providing a comprehensive overview of the current state of AMP research.
To ensure the reliability and validity of the selected studies, a qualitative appraisal was performed using a modified version of the Critical Appraisal Skills Programme (CASP) checklist for systematic reviews (CASP, 2018). This framework was used to assess the methodological quality of each study, considering factors such as clear research objectives, well-defined methodologies, rigorous data analysis and transparent reporting of results. Studies meeting these criteria were prioritised, as they offered high-quality evidence upon which to base the conclusions drawn in the review.
The extracted data were then synthesised through a thematic analysis, identifying common patterns, trends and knowledge gaps. Key areas of focus included the antimicrobial mechanisms of AMPs, their synergy with other preservation techniques, their scalability for commercial use and the regulatory challenges to their adoption in the food industry. By utilising this methodology, the review provides a solid foundation for understanding the current landscape of AMP research in non-dairy biological preservation and offers insights into future research directions that could facilitate the widespread application of AMPs in the food industry.
3. Results and Discussion
3.1 Efficacy of Antimicrobial Peptides (AMPs) in Non-Dairy Food Matrices
The review of existing literature underscores the potential of AMPs to enhance food safety and prolong the shelf life of non-dairy food systems. AMPs have demonstrated broad-spectrum antimicrobial activity, effectively targeting pathogens such as Listeria monocytogenes, Escherichia coli and Salmonella spp. in various plant-based food matrices (Li et al., 2024; Chai et al., 2019). For example, studies involving soy milk revealed that AMP treatment led to a reduction in microbial load of Staphylococcus aureus ATCC 25923 and Escherichia coli ATCC 25922 over a 21-day refrigerated storage period, significantly outperforming untreated samples (Ma et al., 2020).
Similarly, AMP treatments have been applied to minimally processed fruits and vegetables, resulting in extended shelf life over a 9-day period when stored under refrigerated conditions (4°C), compared to control groups. In studies on fruits such as pears and mangoes, the antimicrobial peptide Lactobacillus rhamnosus GG was used to target Listeria monocytogenes. These studies showed a significant reduction in microbial load, suggesting that AMPs may prolong the shelf life of these fruits by inhibiting the growth of spoilage microorganisms, potentially extending their freshness by several days under refrigerated conditions. Notably, these improvements were achieved without compromising critical sensory properties such as flavour and texture, which are essential for consumer acceptance (Ranjith et al., 2022; Iglesias et al., 2018).
Another study by Silva and Lidon (2016) reviewed the use of EU-authorised food preservatives—particularly antimicrobial agents—highlighting the regulatory approval and safety of these conventional preservatives. However, they suggested that AMPs offer a more effective alternative. Unlike some chemical preservatives that may alter food quality, AMPs provide targeted microbial control, maintaining product safety while preserving sensory and nutritional qualities. The review further noted that 45 preservatives are regulated for use in the EU, each having undergone rigorous safety assessments. Despite their effectiveness, these chemical agents face growing consumer concerns regarding potential health risks and the desire for more natural alternatives.
In comparison, AMPs are not yet fully regulated for use in food preservation within the EU, primarily due to gaps in scientific data regarding their safety, stability and efficacy in food matrices. As novel ingredients, AMPs require extensive studies on their toxicity, long-term effects and interactions with food components before regulatory approval can be granted. While AMPs have demonstrated significant antimicrobial activity in research, their integration into the food industry is hindered by the lack of a clear regulatory framework. Regulatory bodies such as the European Food Safety Authority (EFSA) are still evaluating AMPs for potential use in food preservation, meaning that AMPs are not yet widely approved for commercial use. Therefore, while AMPs may offer significant advantages over conventional preservatives, their regulatory approval pathway is more complex, involving comprehensive safety evaluations and standardised testing protocols.
Dhayakaran et al. (2016) expanded the scope of AMPs by investigating soy peptides through a high-throughput drug screening assay. The identification of specific soy peptides with significant antimicrobial properties—such as PGTAVFK and IKAFKEATKVDKVVVLWTA—highlighted the potential for plant-based alternatives to traditional antibiotics. This study reinforced the therapeutic potential of soy-derived peptides and underscored their relevance to food preservation, emphasising the need for advanced screening techniques.
Likewise, López-García et al. (2022) provided a comprehensive overview of bioactive compounds derived from food proteins, emphasising the dual role of peptides as antioxidants and antimicrobial agents. Their findings indicated that innovative extraction technologies could enhance the yield and efficacy of these peptides, thus supporting their application in functional foods. This study highlighted the need for further exploration of peptide–food constituent interactions, which is crucial for developing effective biological preservation strategies.
In another study, Zhang et al. (2021a) demonstrated that three natural AMPs exhibited broad-spectrum and rapid antimicrobial activity against spoilage bacteria by permeabilising membranes and interacting with bacterial DNA. These peptides also showed strong anti-biofilm properties and synergistic effects with chemical preservatives, enhancing food storage outcomes across various matrices. Importantly, the peptides were biodegradable by trypsin, reducing potential toxicity concerns. These findings underscore the potential of AMPs as safe, effective and sustainable solutions for improving food preservation.
Teneva and Denev (2023) addressed the pressing global concern of food safety by advocating for natural, bioactive compounds derived from probiotics and plant extracts. Their findings illustrated the potential of these compounds in extending the shelf life of food products while enhancing nutritional value. This review contributed to understanding biological preservation as a viable method for ensuring food safety and quality, aligning with the growing consumer preference for natural ingredients.
AMPs have proven effective in preserving the shelf life of plant-based beverages by maintaining flavour profiles and microbiological stability while significantly reducing microbial counts. In a study using germinated Pisum sativum seeds, peptides were extracted and evaluated for their antimicrobial properties. Peptides extracted on day six (P6:3) exhibited the highest antibacterial activity against Bacillus cereus, while day four peptides (P4) demonstrated the lowest minimum inhibitory concentration. Although no antifungal activity was observed, the purified peptides extended the shelf life of ‘zobo’, a traditional African beverage. Notably, P4:4 at 10% concentration and pH 2.3 received the highest sensory acceptance, with a 72.22% preference after 72 hours of storage. These findings underscore the potential of AMPs, particularly Pisum sativum peptides, as natural and innovative preservation solutions for plant-based and traditional beverages, addressing the growing consumer demand for clean-label products (Sani et al., 2024).
Finally, the recent study by Thuy et al. (2024) focused on the characterisation of bacteriocin-like inhibitory substances produced by probiotics isolated from fermented foods. This research emphasised the urgent need for new antimicrobial agents to combat antimicrobial resistance, showcasing the efficacy of LAB-derived AMPs against multidrug-resistant pathogens. The investigation into the mechanisms of action of these peptides further highlighted their potential as substitutes for conventional antibiotics, which could be explored as biopreservatives in the food industry.
Table 1: Efficacy of AMPs in Non-Dairy Foods
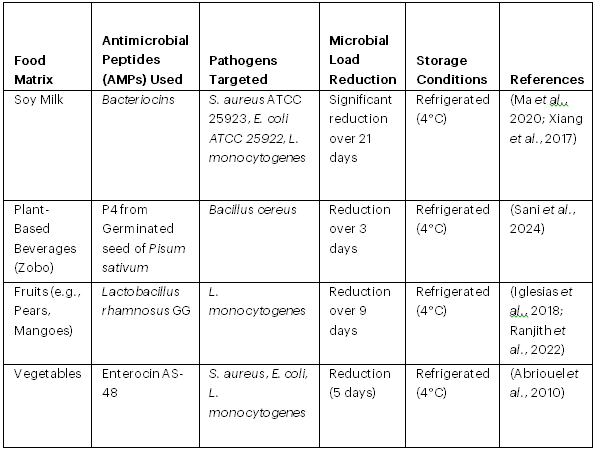
3.2 AMP Stability in Diverse Food Systems
The stability of AMPs is highly dependent on processing conditions and the food matrix into which they are incorporated. Most AMPs retain antimicrobial activity when exposed to mild heat treatments (e.g., up to 60°C). However, at higher temperatures—particularly above 80°C—their efficacy decreases significantly, with studies reporting up to a 40% reduction in antimicrobial activity. This temperature sensitivity could limit the application of AMPs in heat-processed food systems (e.g., pasteurised fruit juices, canned vegetables and UHT-treated beverages) unless strategies such as encapsulation or co-application with heat-resistant preservatives are employed (Xu et al., 2023; Liu et al., 2020).
The pH of the food matrix plays a crucial role in determining AMP stability, as most AMPs are more stable and active in slightly acidic to neutral conditions, which align with the natural pH of many food systems. However, extreme pH levels—such as the high acidity of citrus-based juices or the alkalinity of certain fermented soy products—can destabilise AMPs and reduce their antimicrobial activity. For instance, peptides generally exhibit reduced stability in highly acidic environments (pH < 4), where structural changes may lead to a loss of function (Zapadka et al., 2017).
To address these challenges, recent studies have developed strategies such as encapsulating AMPs in pH-sensitive carriers (e.g., alginate or chitosan nanoparticles) to shield the peptides in acidic environments and facilitate controlled release under neutral conditions. Furthermore, advancements in genetic engineering have produced acid-tolerant AMPs capable of maintaining their stability and antimicrobial activity in low-pH environments. These engineered peptides are particularly suitable for fermented non-dairy foods such as plant-based yoghurts and beverages (Nordström and Malmsten, 2017; Snyder and Worobo, 2014).
The presence of proteolytic enzymes in complex food matrices poses a significant challenge to the stability of AMPs, as these enzymes can degrade the peptides, thereby diminishing their effectiveness as biological preservatives (Ashaolu et al., 2024; Pei et al., 2022). To mitigate this issue, advancements in protective technologies—particularly encapsulation—have gained considerable attention. Encapsulation techniques (e.g., microencapsulation in lipid-based systems, alginate beads or protein-based carriers) not only protect AMPs from enzymatic degradation but also allow for controlled release during storage or consumption, ensuring that the peptides remain active and effective (Singh et al., 2023b; Liu et al., 2021). For example, encapsulating AMPs in alginate–chitosan nanoparticles has been shown to enhance their stability in enzymatic environments while maintaining antimicrobial activity over extended periods (Teixeira et al., 2020).
3.3 Synergistic Effects with Other Preservation Methods
The combination of AMPs with other preservation techniques has been shown to enhance their antimicrobial activity, offering an integrated approach to food safety. Andaluz-Mejía et al. (2022) reported a synergistic reduction in microbial load when AMPs were applied alongside non-thermal technologies such as power ultrasound (US), pulsed electrical fields (PEF) and high hydrostatic pressure (HHP). This approach was more effective than traditional heat treatments alone, particularly in reducing pathogens like Escherichia coli and Listeria monocytogenes.
The synergistic effects of AMPs with other preservation methods have been studied in various non-dairy matrices. For instance, nisin was applied to green juice in combination with thermosonication, resulting in the inactivation of both Gram-positive and Gram-negative bacteria (Oner, 2020). Similarly, cecropin P1 was used in orange juice along with low-power ultrasonication, leading to a reduction in the cell density of E. coli (Fitriyanti and Narsimhan, 2018). Another study demonstrated the application of nisin in fresh apple juice combined with thermosonication, achieving inactivation of naturally occurring microorganisms (Liao et al., 2018). Additionally, natamycin was used in pomegranate juice alongside pasteurisation and sonication, leading to a notable reduction in microbial load (Yikmiş, 2019). These findings underscore the potential of combining AMPs with complementary preservation technologies to enhance food safety and quality.
Moreover, the co-application of AMPs with natural antimicrobials such as organic acids and essential oils not only amplifies their antimicrobial effects but also helps preserve the sensory properties of food. When combined, these agents work synergistically to enhance microbial control while reducing the need for high AMP concentrations, which can sometimes affect flavour or texture. For example, the combination of AMPs with citric acid increased the inhibition of Salmonella enterica by 60%, compared to 30% inhibition when AMP was applied alone (Zuorro et al., 2024). This synergy reduces the required AMP concentrations, thereby lowering production costs while maintaining high efficacy.
In addition, AMP-treated non-dairy products stored under refrigerated conditions consistently maintained microbial stability over extended periods. This preservation approach significantly enhanced both the safety and shelf life of plant-based food products, making AMPs a valuable component in the development of sustainable biological preservation strategies (Meena et al., 2023).
Table 2: Synergistic Effects of AMPs with Other Preservation Methods
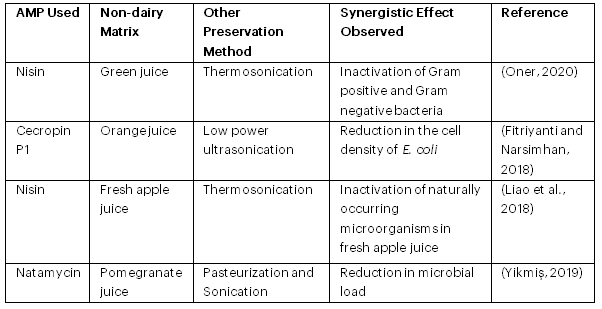
3.4 Challenges in AMP Application
Despite their potential, several challenges impede the widespread adoption of AMPs in non-dairy food preservation. A primary obstacle is the high cost of production, as current manufacturing techniques—including chemical synthesis and recombinant expression—are expensive and not easily scalable for commercial applications. This financial barrier limits the feasibility of integrating AMPs into large-scale food systems (Corrêa et al., 2023). However, recent advancements in production technologies are beginning to address these challenges. Innovations in fermentation-based production, using microorganisms such as yeast or bacteria to express AMPs, have shown promise in lowering costs and improving scalability (Mihooliya and Kumari, 2024). For instance, genetic engineering techniques now allow for more efficient AMP expression in microbial systems, reducing the need for expensive chemical synthesis. Advances in solid-state fermentation and bioreactor design have further improved yields and reduced production time, making AMP production more economically viable for large-scale commercial applications. These developments are expected to enhance the feasibility of AMPs as an affordable and sustainable solution for food preservation, opening up opportunities for their widespread use in non-dairy products (Deo et al., 2022).
Regulatory hurdles further complicate AMP application, with inconsistent approval frameworks across regions and insufficient toxicological data delaying their acceptance as food-grade preservatives. The lack of standardised evaluation criteria for AMPs creates uncertainty for manufacturers, hindering market entry (Santos et al., 2018; Cleveland et al., 2001).
Another significant challenge lies in the interaction of AMPs with complex food matrices, where they can bind to various components such as fats, proteins and carbohydrates, potentially reducing their antimicrobial efficacy. For example, interactions between AMPs and lipids in food products can sequester the peptides, limiting their availability to target and neutralise harmful microorganisms. Similarly, binding to proteins can alter the peptides’ structure, impairing their ability to interact with microbial membranes. These interactions may result in a decrease in the overall activity of AMPs, reducing their effectiveness in preserving food.
To overcome these challenges, researchers are exploring various strategies. One approach involves encapsulating AMPs in protective carriers (e.g., nanoparticles or liposomes) to shield the peptides from interactions with food components and ensure stability during storage. This encapsulation also allows for controlled release, enabling AMPs to gradually act on target pathogens over time. Another potential solution is the design of engineered peptides with modified sequences that are less prone to binding with non-target food components. By optimising the structure of AMPs, their ability to remain active and stable in complex matrices could be enhanced. Additionally, formulating AMPs with synergistic agents—such as essential oils or organic acids—may help mitigate the effects of these interactions by providing broader-spectrum antimicrobial activity while reducing the reliance on high AMP concentrations. Such strategies could enable AMPs to be more effective and practical in preserving non-dairy foods, particularly in products with high fat or protein content where their efficacy is often reduced due to binding or structural modifications (Davoudi et al., 2024; Leon and Segura, 2020).
Addressing these challenges through advancements in cost-effective production methods, comprehensive toxicological studies and tailored formulations is essential for unlocking the full potential of AMPs in biological preservation.
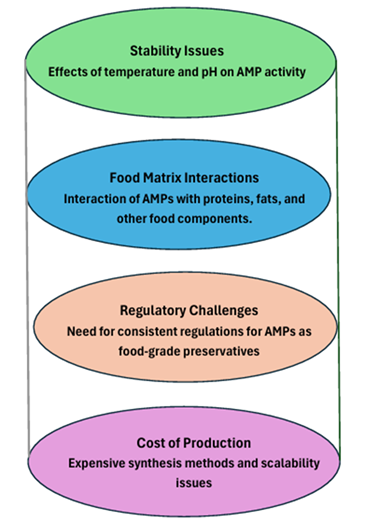
Figure 2: Challenges in the Application of AMPs in Non-Dairy Food Matrices
3.5 Novel Insights and Trends zin Antimicrobial Peptides
Emerging trends in AMP research highlight promising innovations aimed at overcoming existing challenges and expanding their commercial viability. One such innovation is the development of encapsulation technologies—using liposomes and nanoparticles—which protect AMPs from degradation and enhance their stability in complex food matrices. Another promising approach is the engineering of acid- and heat-tolerant AMPs, specifically designed to remain active under the harsh conditions often found in food processing and storage. Additionally, genetically engineered peptides with enhanced stability, tailored antimicrobial activity and improved resistance to degradation in complex food matrices are being developed (Kumari et al., 2024; Singh, 2018). Fermentation-based production methods are also gaining traction as a cost-effective and scalable alternative to traditional synthesis techniques, addressing the economic barriers associated with AMP manufacturing (Zhao et al., 2024; Gollop et al., 2003).
The increasing consumer demand for clean-label, natural food products further supports the adoption of AMPs as sustainable biopreservatives (Ruiz-Ramírez et al., 2024). Their alignment with the global shift towards minimally processed and additive-free foods positions AMPs as an appealing solution for enhancing food safety and shelf life. This trend underscores the potential of AMPs not only to meet market demands but also to contribute to environmental sustainability by reducing food waste through extended product stability (Ruiz-Ramírez et al., 2024; Krishnan et al., 2024; Garin-Murguialday et al., 2024).
Despite these promising developments, optimising AMP stability, scalability and regulatory compliance remains a priority. To facilitate broader adoption in the food industry, regulatory harmonisation is needed whereby global standards for AMP use in food products are developed to streamline the approval process. Regulatory bodies such as the FDA, EFSA and Codex Alimentarius could collaborate to create unified guidelines for AMP safety, efficacy and allowable concentrations in food systems, reducing discrepancies in approval processes across different markets and encouraging wider adoption. Furthermore, revisiting the existing framework for food preservatives to include AMPs in the Generally Recognised as Safe (GRAS) list may enable a faster and more transparent regulatory pathway for AMP inclusion. Collaboration between the food industry and biotechnology companies could also drive the development of cost-effective, scalable production methods for AMPs by leveraging advancements such as recombinant expression systems, fermentation technologies and synthetic biology. Public–private partnerships between academic institutions, government agencies and food companies could support research and development of AMPs with tailored characteristics suited to different food systems, thereby overcoming challenges related to stability, formulation and scaling.
Continued research into innovative production technologies, such as recombinant expression and matrix-specific delivery systems, is essential for realising the full transformative potential of AMPs in non-dairy food systems. These advancements, coupled with collaborative efforts to establish robust regulatory frameworks, could pave the way for their widespread adoption in the global food industry.
4. Discussion
The reviewed studies demonstrate that AMPs possess significant potential to enhance food safety and extend the shelf life of non-dairy food systems. As highlighted in previous research, AMPs exhibit broad-spectrum antimicrobial activity against common foodborne pathogens such as Listeria monocytogenes, Escherichia coli and Salmonella spp. The antimicrobial action of AMPs typically involves several mechanisms that disrupt microbial cell integrity. One primary mechanism is the electrostatic interaction between the positively charged AMPs and the negatively charged components of bacterial cell membranes (e.g., phospholipids). This interaction facilitates the insertion of peptides into the lipid bilayer, resulting in membrane destabilisation, pore formation and eventual cell lysis. In addition to membrane disruption, AMPs can also bind to intracellular targets (e.g., nucleic acids or proteins), thereby inhibiting bacterial cell function and replication. Some AMPs, such as nisin, have been shown to interfere with cell wall biosynthesis, further compromising microbial structural integrity. Furthermore, certain AMPs can trigger oxidative stress by generating reactive oxygen species (ROS), which damage cellular components including lipids, proteins and DNA, ultimately leading to microbial death. These multi-target mechanisms contribute to the broad-spectrum efficacy of AMPs against a wide range of foodborne pathogens, including those typically resistant to traditional chemical preservatives. Understanding these mechanisms allows for the strategic design of AMPs with enhanced antimicrobial properties and stability, thereby optimising their use in food preservation (Xiang et al., 2017). Our findings confirm that AMPs can effectively reduce microbial loads in plant-based products such as soy milk, African traditional beverages like zobo and minimally processed fruits and vegetables, aligning with the results of previous studies (Ma et al., 2020; Sani et al., 2024).
However, while AMPs are effective at reducing microbial load, the extent of their antimicrobial action is influenced by several factors, including food matrix composition and storage conditions. For instance, the presence of fats in food matrices can reduce AMP efficacy by interacting with the peptides and forming complexes that decrease their ability to target microbial membranes. This is particularly evident in high-fat food products like plant-based dairy alternatives or certain meat substitutes. Similarly, the presence of proteins in non-dairy foods (e.g., plant-based beverages) can impact AMP effectiveness by binding to them, altering their conformation and reducing their interaction with pathogens. These interactions highlight the need for tailored AMP formulations and strategies—such as encapsulation or conjugation with other molecules—to overcome matrix-induced challenges and ensure stability and efficacy in diverse food systems (Davoudi et al., 2024).
The potential to maintain the sensory properties of food while extending shelf life is particularly significant for clean-label and minimally processed products, which are increasingly in demand (Xu et al., 2023). Balasubramaniam et al. (2023) report that the global market for clean-label products reached $180 billion in 2020, driven by health, environmental and societal concerns. As consumers increasingly seek natural and transparent food options, biopreservatives like AMPs are gaining traction. Since AMPs are naturally derived, they offer a sustainable and consumer-friendly alternative to traditional chemical preservatives, helping maintain product quality and safety without compromising sensory attributes or nutritional value. Nonetheless, further studies are needed to optimise AMP formulations for various non-dairy matrices, addressing aspects such as the concentration required for optimal antimicrobial activity without negatively impacting sensory properties, the delivery method for improved stability and controlled release, and the overall stability of AMPs under varying environmental conditions (e.g., temperature, pH and the presence of other food components).
The promising synergy between AMPs and other preservation methods—such as non-thermal technologies like high-pressure processing (HPP), pulsed electric field (PEF) processing, ultrasound (US) and natural antimicrobials (e.g., organic acids and essential oils)—can reduce the required AMP concentrations, thereby lowering production costs and enhancing economic feasibility for large-scale application. However, combining these treatments requires careful consideration of potential interactions that might affect AMP stability and activity. For instance, while HPP or PEF can enhance microbial inactivation, they may also adversely affect AMP structure. Future research should investigate optimal conditions for combining these methods and explore advanced techniques like encapsulation to protect AMPs during processing.
Despite the clear potential of AMPs, several challenges must be addressed before they can be widely adopted in non-dairy food systems. The high cost of AMP production remains a significant barrier, although fermentation-based production methods show promise in reducing costs and improving scalability (Rivero-Pino et al., 2023). Optimising microbial strain selection, fermentation conditions and downstream processing (e.g., extraction and purification) is crucial for making AMP production economically viable on a large scale. Regulatory challenges also present a major barrier, as inconsistent approval frameworks, inadequate safety data and limited harmonisation of evaluation criteria delay their acceptance as food-grade preservatives. Regions such as the European Union, the United States and parts of Asia and Africa face different regulatory hurdles, further complicating the global adoption of AMPs (Bose and Sharma, 2025).
Further research on the toxicological safety of AMPs especially for long-term consumption is essential to facilitate regulatory approval. Harmonising guidelines across regions (e.g., through frameworks like the Codex Alimentarius) could streamline approval processes and encourage global adoption of AMP-based preservatives. In addition, a deeper understanding of how AMPs interact with various food components is necessary to develop matrix-specific formulations that maintain efficacy across diverse product types. Emerging trends in AMP research such as advances in nisin variants, genetic engineering for enhanced stability and fermentation-based production methods offer promising solutions to many of these challenges. However, ethical and regulatory implications associated with genetically engineered AMPs require transparent risk assessments, comprehensive safety evaluations and clear labelling to foster public trust (Kumari et al., 2024). The growing demand for clean-label, sustainable food products positions AMPs as key players in the future of food preservation, but further work is needed to ensure their stability, scalability and safety for commercial application.
5. Limitations and Areas for Further Research
While this review has provided valuable insights into the potential and challenges of antimicrobial peptides (AMPs) in non-dairy food systems, some limitations must be acknowledged. First, the studies reviewed predominantly focus on the efficacy of AMPs in laboratory or pilot-scale settings, and more research is needed to assess their performance in real-world, industrial-scale applications. Second, the diversity of AMP types and the variation in food matrices complicate direct comparisons across studies, underscoring the need for standardised testing methods and criteria.
Future research should focus on addressing the gaps in our understanding of AMP interactions with specific food matrices. In addition, investigating the long-term stability and safety of AMPs in various non-dairy food products under commercial processing and storage conditions is crucial for the successful integration of AMPs into biological preservation strategies. Finally, large-scale, multi-centre trials are needed to assess the practical implications of AMP use in food safety and shelf-life extension across different non-dairy food sectors.
Table 3: Knowledge Gaps and Future Research Directions for AMPs in Non-Dairy Biological Preservation
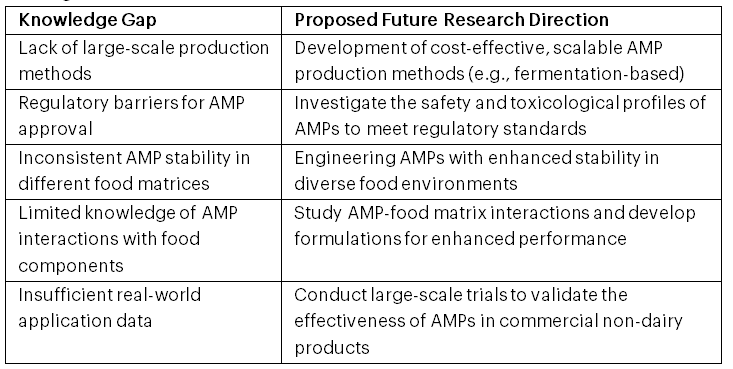
To further advance the field of AMP biological preservation in non-dairy systems, a structured research roadmap is outlined in Figure 3. This roadmap visualises the key stages and future directions for AMPs, from lab-based research to large-scale commercial applications.
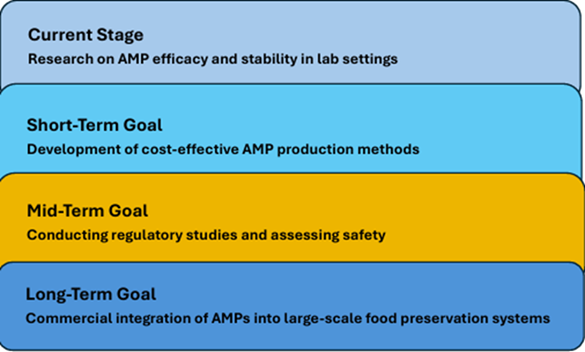
Figure 3: Roadmap for Future Research on AMPs in Non-Dairy Biological Preservation
5. Conclusion
In conclusion, antimicrobial peptides offer significant promise as biological preservatives in non-dairy food systems, with the potential to enhance food safety, extend shelf life, and align with consumer demands for natural and sustainable ingredients. However, challenges such as high production costs, regulatory hurdles, and food matrix interactions must be addressed before AMPs can be widely adopted. Continued advancements in genetic engineering, production technologies and regulatory frameworks, combined with further research into AMP stability and efficacy in diverse food matrices, will be critical for realising the full potential of AMPs in the food industry.
Acknowledgement
The authors have no acknowledgements to report for this study.
Conflict of Interest
The authors declare no conflict of interest in relation to this study.
References
Andaluz-Mejía, L., Ruiz-De Anda, D., & Ozuna, C. (2022). Non-thermal technologies combined with antimicrobial peptides as methods for microbial inactivation: A review. Processes, 10(5), 995–1010. https://doi.org/10.3390/pr10050995
Ashaolu, T. J., Lee, C. C., Ashaolu, J. O., Tarhan, O., Pourjafar, H., & Jafari, S. M. (2024). Pepsin: An excellent proteolytic enzyme for the production of bioactive peptides. Food Reviews International, 40(7), 1875–1912. https://doi.org/ 10.1080/87559129.2023.2238814
Avinash, D., Bisht, A., Kumar, M., Thakur, S., Patel, P., & Kurmi, B. D. (2024). Empowering antimicrobial peptides: Harnessing nanotechnology and engineering strategies to combat microbial resistance. Peptide Science, 24376–24390. https://doi.org/10.1002/pep2.24376
Balasubramaniam, V. M., Lee, J., & Serventi, L. (2023). Understanding new foods: Development of the next generation of food processing, packaging, and ingredients technologies for clean-label foods. In Sustainable Food Innovation (pp. 157–167). Springer International Publishing. https://doi.org/10.1007/978-3-031-12358-0_12
Bose, A., & Sharma, S. (2025). Global regulatory trends and comparative insights: Nutraceuticals in the USA, India, and Europe. PharmaNutrition, 31, 100430–100444. https://doi.org/10.1016/j.phanu.2025.100430
Chai, T. T., Tan, Y. N., Ee, K. Y., Xiao, J., & Wong, F. C. (2019). Seeds, fermented foods, and agricultural by-products as sources of plant-derived antibacterial peptides. Critical Reviews in Food Science and Nutrition, 59(sup1), 162–177. https://doi.org/10.1080/10408398.2018.1561418
Cleveland, J., Montville, T. J., Nes, I. F., & Chikindas, M. L. (2001). Bacteriocins: Safe, natural antimicrobials for food preservation. International Journal of Food Microbiology, 71(1), 1–20. https://doi.org/10.1016/S0168-1605(01)00560-8
Corrêa, J. A. F., de Melo Nazareth, T., Rocha, G. F. D., & Luciano, F. B. (2023). Bioactive antimicrobial peptides from food proteins: Perspectives and challenges for controlling foodborne pathogens. Pathogens, 12(3), 477–496. https://doi.org/10.3390/pathogens12030477
Critical Appraisal Skills Programme. (2018). CASP Systematic Review Checklist. Retrieved from https://casp-uk.net/casp-tools-checklists/systematic-review-checklist/
Davoudi, M., Gavlighi, H. A., Javanmardi, F., Benjakul, S., & Nikoo, M. (2024). Antimicrobial peptides derived from food byproducts: Sources, production, purification, applications, and challenges. Comprehensive Reviews in Food Science and Food Safety, 23(5), e13422–e13437. https://doi.org/10.1111/1541-4337.13422
Deo, S., Turton, K. L., Kainth, T., Kumar, A., & Wieden, H. J. (2022). Strategies for improving antimicrobial peptide production. Biotechnology Advances, 59, 107968–107982. https://doi.org/10.1016/j.biotechadv.2022.107968
Devi, R., Sharma, E., Thakur, R., Lal, P., Kumar, A., Altaf, M. A., & Kumar, R. (2023). Non-dairy prebiotics: Conceptual relevance with nutrigenomics and mechanistic understanding of the effects on human health. Food Research International, 170, 112980. https://doi.org/10.1016/j.foodres.2023.112980.
Dhayakaran, R., Neethirajan, S., & Weng, X. (2016). Investigation of the antimicrobial activity of soy peptides by developing a high-throughput drug screening assay. Biochemistry and Biophysics Reports, 6, 149–157. https://doi.org/10.1016/j.bbrep.2016.04.001
Erdem Büyükkiraz, M., & Kesmen, Z. (2022). Antimicrobial peptides (AMPs): A promising class of antimicrobial compounds. Journal of Applied Microbiology, 132(3), 1573–1596. https://doi.org/10.1111/jam.15314
Fitriyanti, M., & Narsimhan, G. (2018). Synergistic effect of low-power ultrasonication on antimicrobial activity of cecropin P1 against E. coli in food systems. LWT, 96, 175–181. https://doi.org/10.1016/j.lwt.2018.05.016
Garin-Murguialday, N., Espina, L., Virto, R., & Pagán, R. (2024). Lactic acid bacteria and Bacillus subtilis as potential protective cultures for biopreservation in the food industry. Applied Sciences, 14(10), 4016–4029. https://doi.org/10.3390 /app14104016
Gollop, N., Toubia, D., Shushan, G. B., & Zakin, V. (2003). High production system of the antibacterial peptide PLG‐1. Biotechnology Progress, 19(2), 436–439. https://doi.org/10.1021/bp025668r
Guilhelmelli, F., Vilela, N., Albuquerque, P., Derengowski, L. D. S., Silva-Pereira, I., & Kyaw, C. M. (2013). Antibiotic development challenges: The various mechanisms of action of antimicrobial peptides and of bacterial resistance. Frontiers in Microbiology, 4(353), 1–12. https://doi.org/10.3389/fmic b.2013.00353
Harshitha, T., Dasalkar, A. H., Parinitha, A., & Yannam, S. K. (2024). Food additives and preservatives. In Frontiers in Food Biotechnology (pp. 249–282). Springer Nature Singapore. https://doi.org/10.1007/978-981-97-3261-6_15
Hidalgo-Fuentes, B., de Jesús-José, E., Cabrera-Hidalgo, A. D. J., Sandoval-Castilla, O., Espinosa Solares, T., González-Reza, R. M., & Aguilar-Toalá, J. E. (2024). Plant-based fermented beverages: Nutritional composition, sensory properties, and health benefits. Foods, 13(6), 844–863. https://doi.org/10 .3390/foods13060844
Horlacher, N., Oey, I., & Agyei, D. (2023). Learning from tradition: Health-promoting potential of traditional lactic acid fermentation to drive innovation in fermented plant-based dairy alternatives. Fermentation, 9(5), 452.
Iglesias, M. B., Echeverría, G., Viñas, I., López, M. L., & Abadias, M. (2018). Biopreservation of fresh-cut pear using Lactobacillus rhamnosus GG and effect on quality and volatile compounds. LWT, 87, 581–588. https://doi.org/10. 1016/j.lwt.2017.09.025
Kapetanakou, A. E., Passiou, K. E., Chalkou, K., & Skandamis, P. N. (2021). Assessment of spoilage potential posed by Alicyclobacillus spp. in plant-based dairy beverages mixed with fruit juices during storage. Journal of Food Protection, 84(3), 497–508. https://doi.org/10.4315/JFP-20-298
Karbowiak, M., Szymański, P., & Zielińska, D. (2023). Synergistic effect of combination of various microbial hurdles in the biopreservation of meat and meat products—A systematic review. Foods, 12(7), 1430. https://doi.org/10 .3390/foods12071430
Krishnan, S. V., Nampoothiri, K. M., Suresh, A., Linh, N. T., Balakumaran, P. A., Pócsi, I., & Pusztahelyi, T. (2024). Fusarium biocontrol: Antagonism and mycotoxin elimination by lactic acid bacteria. Frontiers in Microbiology, 14, 1260166. https://doi.org/10.3389/fmicb.2023.1260166
Kumar, P., Singh, S., Sankhyan, S., & Ray, S. (2024). Metabolic engineering of lactic acid bacteria for antimicrobial peptide production. In Antimicrobial Peptides from Lactic Acid Bacteria: Diversity, Biosynthesis and Applications (pp. 67–95). Springer Nature Singapore. https://doi.org/10.1007/978-981-97-3413-9_3
Kumari, D., Chaudhary, P., & Janmeda, P. (2024). Genetic engineering and designed promising preservative in food products. In A. Prakash & A. Kuila (Eds.), Nonthermal Food Processing, Safety, and Preservation (pp. 299–324). Wiley. https://doi.org/10.1002/9781394186631.ch16
Leon, M. A., & Segura Campos, M. R. (2020). Review of antimicrobial peptides as promoters of food safety: Limitations and possibilities within the food industry. Journal of Food Safety, 40(6), 12854–12569. https://doi.org/10.1111/jfs.12854
Li, S., Jiang, S., Jia, W., Guo, T., Wang, F., Li, J., & Yao, Z. (2024). Natural antimicrobials from plants: Recent advances and future prospects. Food Chemistry, 432, 137231. https://doi.org/10.1016/j.foodchem.2023.137231
Liao, H., Jiang, L., Cheng, Y., Liao, X., & Zhang, R. (2018). Application of nisin-assisted thermosonication processing for preservation and quality retention of fresh apple juice. Ultrasonics Sonochemistry, 42, 244–249. https://doi.org/ 10.1016/j.ultsonch.2017.11.020
Liu, S., Brul, S., & Zaat, S. A. (2020). Bacterial persister cells and spores in the food chain: Their potential inactivation by antimicrobial peptides (AMPs). International Journal of Molecular Sciences, 21(23), 8967–8981. https://doi.org/ 10.3390/ijms21238967
Liu, Y., Sameen, D. E., Ahmed, S., Dai, J., & Qin, W. (2021). Antimicrobial peptides and their application in food packaging. Trends in Food Science & Technology, 112, 471–483. https://doi.org/10.1016/j.tifs.2021.04.019
López-García, G., Dublan-García, O., Arizmendi-Cotero, D., & Gómez Oliván, L. M. (2022). Antioxidant and antimicrobial peptides derived from food proteins. Molecules, 27(4), 1343–1374. https://doi.org/10.3390/molecules27041343
Luo, Y., & Song, Y. (2021). Mechanism of antimicrobial peptides: Antimicrobial, anti-inflammatory and antibiofilm activities. International Journal of Molecular Sciences, 22(21), 11401–11419. https://doi.org/10.3390/ijms222111401
Ma, J., Yu, W., Hou, J., Han, X., Shao, H., & Liu, Y. (2020). Characterisation and production optimisation of a broad-spectrum bacteriocin produced by Lactobacillus casei KLDS 1.0338 and its application in soybean milk biopreservation. International Journal of Food Properties, 23(1), 677–692. https://doi.org/10.1080/10942912.2020.1751656
Mäkinen, O. E., Wanhalinna, V., Zannini, E., & Arendt, E. K. (2016). Foods for special dietary needs: Non-dairy plant-based milk substitutes and fermented dairy-type products. Critical Reviews in Food Science and Nutrition, 56(3), 339–349. https://doi.org/10.1080/10408398.2012.761950
McClements, D. J., Das, A. K., Dhar, P., Nanda, P. K., & Chatterjee, N. (2021). Nanoemulsion-based technologies for delivering natural plant-based antimicrobials in foods. Frontiers in Sustainable Food Systems, 5, 643208. https://doi.org/10.3389/fsufs.2021.643208
Meena, K. K., Taneja, N. K., Jain, D., Ojha, A., Saravanan, C., & Bunkar, D. S. (2023). Spontaneously fermented cereal-based products: An ancient health-promoting formula for consumption of probiotic lactic acid bacteria. Biointerface Research in Applied Chemistry, 13(5), 465–491. https://doi.org/10.33263 /BRIAC135.465
Mihooliya, K. N., & Kumari, A. (2024). Bioprocessing and market aspects of antimicrobial peptides. In Evolution of Antimicrobial Peptides: From Self-Defense to Therapeutic Applications (pp. 167–197). Springer Nature Switzerland. https://doi.org/10.1007/978-3-031-67515-7_7
Mishra, T., Machireddy, J., & Vuppu, S. (2024). Comprehensive study on hygiene and quality assessment practices in the production of drinkable dairy-based and plant-based fermented products. Fermentation, 10(9), 489–510. https://doi.org/10.3390/fermentation10090489
Moravej, H., Moravej, Z., Yazdanparast, M., Heiat, M., Mirhosseini, A., Moosazadeh Moghaddam, M., & Mirnejad, R. (2018). Antimicrobial peptides: Features, action and their resistance mechanisms in bacteria. Microbial Drug Resistance, 24(6), 747–767. https://doi.org/10.1089/mdr.2017.0392
Nongonierma, A. B., & FitzGerald, R. J. (2016). Strategies for the discovery, identification and validation of milk protein-derived bioactive peptides. Trends in Food Science & Technology, 50, 26–43. https://doi.org/10.1016/j.tifs. 2016.01.022
Nordström, R., & Malmsten, M. (2017). Delivery systems for antimicrobial peptides. Advances in Colloid and Interface Science, 242, 17–34. https://doi.org/10.1016 /j.cis.2017.01.005
Novais, C., Molina, A. K., Abreu, R. M., Santo-Buelga, C., Ferreira, I. C., Pereira, C., & Barros, L. (2022). Natural food colourants and preservatives: A review, a demand, and a challenge. Journal of Agricultural and Food Chemistry, 70(9), 2789–2805. https://doi.org/10.1021/acs.jafc.1c07533
Obahiagbon, E. G., & Ogwu, M. C. (2024). Organic food preservatives: The shift towards natural alternatives and sustainability in the Global South’s markets. In Food Safety and Quality in the Global South (pp. 299–329). Springer Nature Singapore. https://doi.org/10.1007/978-981-97-2428-4_10
Oner, M. E. (2020). The effect of high-pressure processing or thermosonication in combination with nisin on microbial inactivation and quality of green juice. Journal of Food Processing and Preservation, 44(10), e14830–e14844. https://doi.org/10.1111/jfpp.14830
Pandey, S., Sharma, K., & Gundabala, V. (2022). Antimicrobial bio-inspired active packaging materials for shelf life and safety development: A review. Food Bioscience, 48, 101730. https://doi.org/10.1016/j.fbio.2022.101730
Patil, P. J., Usman, M., Zhang, C., Mehmood, A., Zhou, M., Teng, C., & Li, X. (2022). An updated review on food-derived bioactive peptides: Focus on the regulatory requirements, safety, and bioavailability. Comprehensive Reviews in Food Science and Food Safety, 21(2), 1732–1776. https://doi.org/10.1111/1541-4337.12911
Pei, J., Gao, X., Pan, D., Hua, Y., He, J., Liu, Z., & Dang, Y. (2022). Advances in the stability challenges of bioactive peptides and improvement strategies. Current Research in Food Science, 5, 2162–2170. https://doi.org/10.1016/j.crfs. 2022.10.031
Ranjith, F. H., Muhialdin, B. J., Arroo, R., Yusof, N. L., Mohammed, N. K., & Hussin, A. S. M. (2022). Lacto-fermented polypeptides integrated with edible coatings for mango (Mangifera indica L.) biopreservation. Food Control, 134, 108708. https://doi.org/10.1016/j.foodcont.2021.108708
Rasika, D. M. D., Vidanarachchi, J. K., Luiz, S. F., Azeredo, D. R. P., Cruz, A. G., & Ranadheera, C. S. (2021). Probiotic delivery through non-dairy plant-based food matrices. Agriculture, 11(7), 599–621. https://doi.org/10.3390/agriculture 11070599
Rivero-Pino, F., Leon, M. J., Millan-Linares, M. C., & Montserrat-De la Paz, S. (2023). Antimicrobial plant-derived peptides obtained by enzymatic hydrolysis and fermentation as components to improve current food systems. Trends in Food Science & Technology, 135, 32–42. https://doi.org/10.1016/j.tifs.2023.03.005
Ruiz-Ramírez, Y., Guadarrama-Mendoza, P. C., & Valadez-Blanco, R. (2024). Antimicrobial peptides from lactic acid bacteria as a hurdle technology component for food preservation: Applications and safety considerations. In S. Ray, P. Kumar, & M. Mandal (Eds.), Antimicrobial Peptides from Lactic Acid Bacteria (pp. 339–368). Springer. https://doi.org/10.1007/978-981-97-3413-9_13
Sabaté, J., & Soret, S. (2014). Sustainability of plant-based diets: Back to the future. The American Journal of Clinical Nutrition, 100, 476S–482S. https://doi.org/10. 3945/ajcn.113.071522
Sampaio de Oliveira, K. B., Leite, M. L., Rodrigues, G. R., Duque, H. M., da Costa, R. A., Cunha, V. A., & Dias, S. C. (2020). Strategies for recombinant production of antimicrobial peptides with pharmacological potential. Expert Review of Clinical Pharmacology, 13(4), 367–390. https://doi.org/10.1080/17512433. 2020.1764347
Sani, H. M., Bukar, A., & Magashi, A. M. (2024). Preservative properties of antimicrobial peptides of germinated seeds of Pisum sativum (garden pea) on roselle flowers (Hibiscus sabdarifa L.) drink. UMYU Scientifica, 3(4), 134–147.
Santos, J. C., Sousa, R. C., Otoni, C. G., Moraes, A. R., Souza, V. G., Medeiros, E. A., & Soares, N. F. (2018). Nisin and other antimicrobial peptides: Production, mechanisms of action, and application in active food packaging. Innovative Food Science & Emerging Technologies, 48, 179–194. https://doi.org/10.1016 /j.ifset.2018.06.008
Sharma, N., Maibam, B. D., & Sharma, M. (2024). Review on the effect of innovative technologies on shelf-life extension of non-dairy sources from plant matrices. Food Chemistry Advances, 100781, 1–15. https://doi.org/10.1016 /j.focha.2024.100781
Shwaiki, L. N., Arendt, E. K., & Lynch, K. M. (2022). Plant compounds for the potential reduction of food waste – A focus on antimicrobial peptides. Critical Reviews in Food Science and Nutrition, 62(15), 4242–4265. https://doi. org/10.1080/10408398.2021.1873733
Silva, M. M., & Lidon, F. C. (2016). Food preservatives—An overview on applications and side effects. Emirates Journal of Food and Agriculture, 28(6), 366–373. Retrieved from https://pdfs.semanticscholar.org/7d3f/cf6896f938cab2639 1a2541c2ecada647fd2.pdf
Singh, A., Du, R. T., Wandhare, A. G., Sian, J. K., Singh, B. P., Sihag, M. K., & Panwar, H. (2023a). Milk-derived antimicrobial peptides: Overview, applications, and future perspectives. Probiotics and Antimicrobial Proteins, 15(1), 44–62. https://doi.org/10.1007/s12602-022-10004-y
Singh, B. P., Rohit, Manju, K. M., Sharma, R., Bhushan, B., Ghosh, S., & Goel, G. (2023b). Nano-conjugated food-derived antimicrobial peptides as natural biopreservatives: A review of technology and applications. Antibiotics, 12(2), 244–262. https://doi.org/10.3390/antibiotics12020244
Singh, V. P. (2018). Recent approaches in food bio-preservation—a review. Open Veterinary Journal, 8(1), 104–111. Retrieved from https://www.ajol.info /index.php/ovj/article/view/168933
Snyder, A. B., & Worobo, R. W. (2014). Chemical and genetic characterisation of bacteriocins: Antimicrobial peptides for food safety. Journal of the Science of Food and Agriculture, 94(1), 28–44. https://doi.org/10.1002/jsfa.6293
Teixeira, M. C., Carbone, C., Sousa, M. C., Espina, M., Garcia, M. L., Sanchez-Lopez, E., & Souto, E. B. (2020). Nanomedicines for the delivery of antimicrobial peptides (AMPs). Nanomaterials, 10(3), 560–582. https://doi.org/10.3390 /nano10030560
Teneva, D., & Denev, P. (2023). Biologically active compounds from probiotic microorganisms and plant extracts used as biopreservatives. Microorganisms, 11(8), 1896–1912. https://doi.org/10.3390/microorganisms11081896
Thuy, T. T. D., Lu, H. F., Bregente, C. J. B., Huang, F. C. A., Tu, P. C., & Kao, C. Y. (2024). Characterisation of the broad-spectrum antibacterial activity of bacteriocin-like inhibitory substance-producing probiotics isolated from fermented foods. BMC Microbiology, 24(1), 85–99. https://doi.org/1 0.1186/s12866-024-03245-0
Tkaczewska, J. (2020). Peptides and protein hydrolysates as food preservatives and bioactive components of edible films and coatings—a review. Trends in Food Science & Technology, 106, 298–311. https://doi.org/10.1016/j.tifs.2020.10.022
Wibowo, D., & Zhao, C. X. (2019). Recent achievements and perspectives for large-scale recombinant production of antimicrobial peptides. Applied Microbiology and Biotechnology, 103, 659–671. https://doi.org/10.1007/s00253-018-9524-1
Xiang, N., Lyu, Y., Zhu, X., Bhunia, A. K., & Narsimhan, G. (2017). Effect of physicochemical properties of peptides from soy protein on their antimicrobial activity. Peptides, 94, 10–18. https://doi.org/10.1016/j.peptides.2017.05.010
Xu, S., Tan, P., Tang, Q., Wang, T., Ding, Y., Fu, H., & Ma, X. (2023). Enhancing the stability of antimicrobial peptides: From design strategies to biomedical applications. Chemical Engineering Journal, 475, 145923. https://doi.org/ 10.1016/j.cej.2023.145923
Yikmiş, S. (2019). Investigation of the effects of non-thermal, combined and thermal treatments on the physicochemical parameters of pomegranate (Punica granatum L.) juice. Food Science and Technology Research, 25(3), 341–350. https://doi.org/10.3136/fstr.25.341
Zapadka, K. L., Becher, F. J., Gomes dos Santos, A. L., & Jackson, S. E. (2017). Factors affecting the physical stability (aggregation) of peptide therapeutics. Interface Focus, 7(6), 20170030–20170047. https://doi.org/10.1098 /rsfs.2017.0030
Zhang, F., Zhang, M., Chen, Y., Ouyang, J., Wang, Y., Yang, H., & Wang, Y. (2021a). Antimicrobial, anti-biofilm properties of three naturally occurring antimicrobial peptides against spoilage bacteria, and their synergistic effect with chemical preservatives in food storage. Food Control, 123, 107729. https://doi.org/10. 1016/j.foodcont.2020.107729
Zhang, Q. Y., Yan, Z. B., Meng, Y. M., Hong, X. Y., Shao, G., Ma, J. J., & Fu, C. Y. (2021b). Antimicrobial peptides: Mechanism of action, activity and clinical potential. Military Medical Research, 8, 1–25. https://doi.org/10.1186/s40779-021-00343-2
Zhao, L., Liu, X., Wang, S., Yin, Z., An, T., Zhang, J., & Liu, Y. (2024). Research progress on fermentation-produced plant-derived bioactive peptides. Frontiers in Pharmacology, 15, 1438947. https://doi.org/10.3389/fphar.2024.1438947
Zheng, Y., Du, Y., Qiu, Z., Liu, Z., Qiao, J., Li, Y., & Caiyin, Q. (2022). Nisin variants generated by protein engineering and their properties. Bioengineering, 9(6), 251–264. https://doi.org/10.3390/bioengineering9060251
Zuorro, A., Lavecchia, R., Contreras-Ropero, J. E., Martínez, J. B. G., Barajas-Ferreira, C., & Barajas-Solano, A. F. (2024). Natural antimicrobial agents from algae: Current advances and future directions. International Journal of Molecular Sciences, 25(21), 11826–11846.
About this Article
Cite this Article
APA
Popoola O. A., Agarry O. O. & Orukotan A. A.(2025). Antimicrobial Peptides in Non-Dairy Matrices: Innovations and Challenges in Biological Preservation. In Akinyele B.J., Kayode R. & Akinsemolu A.A. (Eds.), Microbes, Mentorship, and Beyond: A Festschrift in Honour of Professor F.A. Akinyosoye. SustainE
Chicago
Popoola O. A., Agarry O. O. and Orukotan A. A. 2025. “Antimicrobial Peptides in Non-Dairy Matrices: Innovations and Challenges in Biological Preservation.” In Microbes, Mentorship, and Beyond: A Festschrift in Honour of Professor F.A. Akinyosoye, edited by Akinyele B.J., Kayode R. and Akinsemolu A.A., SustainE.
Received
15 December 2024
Accepted
10 January 2025
Published
4 February 2025
Corresponding Author Email: atinuke.solina@gmail.com
Disclaimer: The opinions and statements expressed in this article are the authors’ sole responsibility and do not necessarily reflect the viewpoints of their affiliated organizations, the publisher, the hosted journal, the editors, or the reviewers. Furthermore, any product evaluated in this article or claims made by its manufacturer are not guaranteed or endorsed by the publisher.
Distributed under Creative Commons CC-BY 4.0
Share this article
Use the buttons below to share the article on desired platforms.